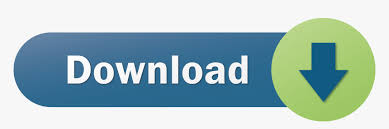
Kings Edit 1.xx serial key or number
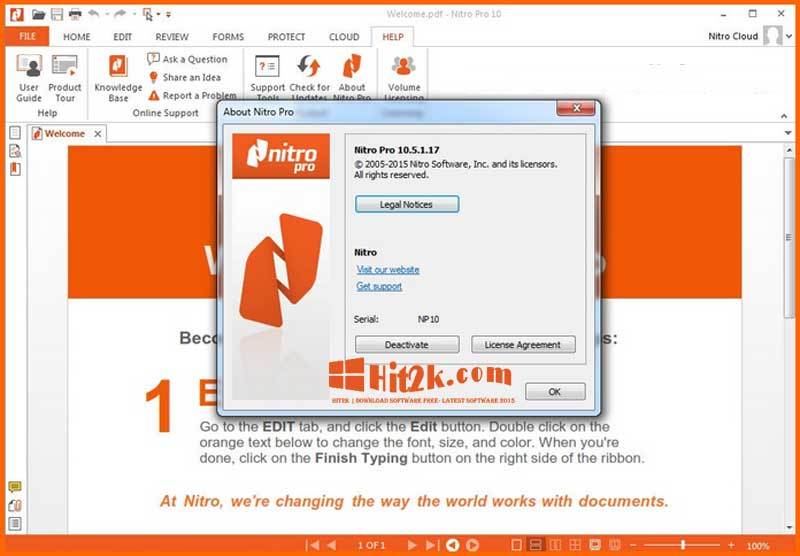
Kings Edit 1.xx serial key or number
Security Warrior by Cyrus Peikari, Anton Chuvakin
In the previous chapters, we covered reverse engineering on traditional platforms such as Win32 and Linux. However, what about the little guys? Can you reverse engineer software on embedded operating systems? Why would you want to?
Many embedded operating systems are stripped-down microversions of their big brothers. An embedded operating system brings the power of a complete OS to small devices such as mobile phones or watches, which suffer from severely restricted processing and memory resources. However, as embedded devices continue to increase in sophistication, their vulnerability to attack increases as well. Already the first computer viruses have hit embedded platforms, as we describe in Chapter 17. Corporate spyware will likely follow soon. With hundreds of millions of “smart” consumer appliances on the horizon, the potential for abuse keeps increasing.
Embedded RCE is still in its infancy. In this chapter, we introduce embedded OS architecture and how to crack the applications that run on it. For our example, we have chosen Windows CE, which powers many Windows Mobile OS flavors such as PocketPC and Smartphone. Windows CE is a semi-open, scalable, 32-bit, true-multitasking operating system that has been designed to run with maximum power on minimum resources. This OS is actually a miniature version of Windows 2000/XP that can run on appliances as small as a watch.
Why have we chosen Windows CE for our reverse engineering research, instead of friendly, open source, and free embedded Linux? For better or worse, CE is set to become one of the most prevalent operating systems of all time, thanks to aggressive marketing tactics by Microsoft. In addition, because of their closed nature, Windows platforms usually see the majority of viruses and unethical corporate spyware. Thus, the need to reverse engineer embedded Windows applications is more pressing. Download the free eMbedded Visual Tools (MVT) package from Microsoft.com and get cracking—literally.
Windows CE is the basis of all Windows Mobile PocketPC and Smartphone devices. In addition, using the CE Platform Builder, any programmer can create her own miniature operating system based on Windows CE. Consequently, CE is starting to control a vast array of consumer devices, ranging from toasters to exercise bicycles. Because of its growing prevalence, if you want to become proficient at reverse engineering applications on mobile devices it is important to understand the basics of how this operating system works. This segment briefly covers the Windows CE architecture, with a deeper look at topics important to understand when reversing.
In the world of miniature gadgets, physics is often the rate-limiting step. For example, the intense heat generated by high-speed processors in notebook PCs has been shown to be hot enough to fry eggs. In fact, News.com reported that one unfortunate man inadvertently burned his genitals with a laptop computer (http://www.news.com.au/common/story_page/0,4057,5537960%255E1702,00.html)!
Windows CE devices are likewise limited in their choice of processors. The following is a list of processors supported by Windows CE:
- ARM
Supported processors include ARM720T, ARM920T, ARM1020T, StrongARM, and XScale. ARM-based processors are by far the most common choice of CE devices at the time of this writing.
- MIPS
Supported processors include MIPS II/32 w/FP, MIPS II/32 w/o FP, MIPS16, MIPS IV/64 w/FP, and MIPS IV/64 w/o FP.
- SHx
Supported processors include SH-3, SH-3 DSP, and SH-4.
- x86
Supported processors include 486, 586, Geode, and Pentium I/II/III/IV.
If heat dissipation is a serious issue, the best choice is one of the non-x86 processors that uses a reduced level of power. The reduction in power consumption reduces the amount of heat created during processor operation, but it also limits the processor speed.
Kernel, Processes, and Threads
The kernel is the key component of a Windows CE OS. It handles all the core functions of the OS, such as processes, threads, and memory management. It also handles scheduling and interrupts. However, it is important to understand that Windows CE uses parts from its big brother—i.e., desktop Windows software. This means its threading, processing, and virtual memory models are similar to those of traditional Windows platforms.
While CE has a lot in common with traditional Windows, there are several items that distinguish it. These differences center on the use of memory and the simple fact that there is no hard drive (as discussed in the next section). In addition, dynamic link libraries (DLLs) in Windows CE are not implemented as they are in other Windows operating systems. Instead, they are used in such a way as to maximize the available memory. Integrating them into the core operating system means that DLLs don’t take up precious space when they are executed. This is an important concept to understand before trying to reverse a program in Windows CE. Due to this small difference, attempting to break a program while it is executing a system DLL is not allowed by Microsoft’s MVT.
A process in Windows CE represents an executing program. The number of processes is limited to 32, but each process can execute a theoretically unlimited number of threads. Each thread has a 64K memory block assigned to it, in addition to an ID and a set of registers. It is important to understand this concept because when debugging a program, you will be monitoring the execution of a particular thread, its registers, and the allotted memory space. In the process, you will be able to deduce hidden passwords, serial numbers, and more.
Processes can run in two modes: kernel and user. A kernel process has direct access to the OS and the hardware. This gives it more power, but a crash in a kernel process often crashes the whole OS. A user process, on the other hand, operates outside the kernel memory—but a crash only kills the running program, not the whole OS. In Windows CE, any third-party program will operate in user mode, which means it is protected. In other words, if you crash a program while reversing it, the whole OS will not crash (though you still may need to reboot the device).
There are two other important points to understand. First, one process cannot affect the data of another process. While related threads can interact with each other, a process is restricted to its own memory slot. The second point to remember is that each existing thread is continuously being stopped and restarted by a scheduler (discussed next). This is how multitasking is actually performed. While it may appear that more than one program is running at a time, the truth is that only one thread may execute at any one time on single-processor devices.
The scheduler is responsible for managing the thread process times. It does this by giving each thread a chance to use the processor. By continuously moving from thread to thread, the scheduler ensures that each gets a turn. Three key features for adjusting processor time are built into the scheduler.
The first feature is a method that is used to increase the amount of processor time. The secret is found in multithreading an application. Since the scheduler assigns processor time at the thread level, a process with 10 threads will get 10 times the processor time of a process with one thread.
Another method for gaining more processor time is to increase the process priority; but it’s not encouraged unless necessary. Changing priority levels can cause serious problems in other programs, and it affects the speed of the computing device as a whole. The THREAD_PRIORITY_TIME_CRITICAL priority is important; it forces the processor to complete the critical thread.
The final interesting feature of the scheduler deals with a problem that can arise when priority threading is used. If a low-priority thread is executing and it ties up a resource needed by a higher-priority thread, the system could become unstable. In short, a paradox is created in which the high thread waits for the low thread to finish, which in turn waits on the high to complete. To prevent this situation from occurring, the scheduler will detect such a paradox and boost the lower-priority thread to a higher level, thus allowing it to finish.
Note that all of these problems are issues that every Windows OS must deal with. A Windows Mobile device may seem different, but it is still a Microsoft product, and as such it is limited by those products’ common constraints.
One of the unique properties of most devices running Windows CE is the lack of a disc hard drive. Instead of spinning discs, pocket PCs use old-fashioned RAM (Random Access Memory) and ROM (Read Only Memory) to store data. While this may seem like a step back in technology, the use of static memory like ROM is on the rise and will eventually make moving storage devices obsolete. The next few paragraphs explain how memory in a Windows CE device is used to facilitate program execution.
In a Windows CE device, the entire operating system is stored in ROM. This type of memory is typically read-only and is not used to store temporary data that can be deleted. On the other hand, data in RAM is constantly being updated and changed. This memory is used to hold all files and programs that are loaded into the Windows CE-based device.
RAM is also used to execute programs. When a third-party game is executed, it is first copied into RAM and is executed from there. This is why a surplus of RAM is important in a Windows CE device. However, the real importance of RAM is that its data can be written to and accessed by an address. This is necessary because a program will often have to move data around. Since each program is allotted a section of RAM to run in when it is executed, it must be able to write directly to its predefined area.
While ROM is typically only used as a static storage area, in Windows CE it can be used to execute programs. This process is known as Execute In Place (XIP). In other words, RAM is not required to hold the ROM’s data as a program executes. This freedom allows RAM to be used for other important applications. However, it only works with ROM data that is not compressed. While compression allows more data to be stored in ROM, the decompression will force any execution to be done via RAM.
RAM usage on a Windows CE device is divided between two functions. The first is the object store, which is used to hold files and data that are used by the programs but are not stored in ROM. In particular, the object store holds compressed program files, user files, database files, and the infamous Windows registry file. Although this data is stored in RAM, it remains intact when the device is turned off, because the RAM is kept charged by the power supply. This is the reason it is very important to never let the charge on a Pocket PC device completely die. If this happens, the RAM loses power and resets. It dumps all installed programs and wipes everything on the device except what is stored in ROM. This is referred to as a hard reboot when dealing with a Pocket PC device.
The second function of the RAM is to facilitate program execution. As previously mentioned, when a program is running, it needs to store the information it is using—this is the same function that RAM serves on a typical desktop PC. Any data passing through a program, such as a password or serial number, will be written to the RAM at one time or another.
Windows CE does have a limit on the RAM size. In Windows CE 3.0 it is 256 MB with a 32 MB limit on each file, but in Windows CE .NET this value has been increased to a rather large 4 GB. In addition, there is a limit to the number of files that can be stored in RAM (4 million) and to the number of programs that can operate at the same time. This brings us to multitasking.
Windows CE was designed to be a true multitasking operating system. Just like other modern Windows operating systems, it allows more than one program to be open at a time. In other words, you can listen to an MP3 while taking notes and checking out sites on the Internet. Without multitasking, you would be forced to close one program before opening another. However, you must be careful not to open too many programs on a Windows CE device. Since you are limited by the amount RAM in the device, and each open program takes up a chunk of the RAM, you can quickly run out of memory.
Finally, the limitation of RAM in a pocket PC also affects the choice of operating system. Since Windows CE devices may only have 32-128 MB of internal RAM, they do not make good platforms for operating systems that use a lot of memory, such as embedded Windows XP. In this OS, the minimum footprint for a program is 5 MB. On the other hand, Windows CE only requires 200K; this is a 2500% difference.
Graphics, Windowing, and Event Subsystem (GWES)
This part of the Windows CE architecture is responsible for handling all the input (e.g., stylus) and output (e.g., screen text and images). Since every program uses windows to receive messages, it is a very important part of Windows CE. It is one of the areas you need to understand to successfully reverse a program.
Without going into too much detail, you should know that every Windows CE process is assigned its own windows messaging queue. The queue is similar to a stack of papers that is added to and read from. This queue is created when the program calls GetMessage, which is very common in Windows CE programs. While the program executes and interacts with the user, messages are placed in and removed from the queue. The following is a list and explanation of the common commands that you will see while reverse engineering:
- PostMessage
Places message on queue of target thread, which is returned immediately to the process/thread
- SendMessage
Places message on queue, but does not return until it is processed
- SendThreadMessage
Sends messages directly to thread instead of to queue
These Message commands, and others, act as bright, virtual flares when reversing a program. For example, if a “Sorry, wrong serial number” warning is flashed on the screen, you can bet some Message command was used. By looking for the use of this command in a disassembler, you can find the part of the program that needs further research.
We’ve given you a quick inside look at how Windows CE operates. This information is required reading for the rest of the chapter. Understanding processing, memory architecture, and how Windows CE uses messages to communicate with the executing program will make it easier for you to understand how CE cracking works. Just as a doctor must understand the entire human body before diagnosing even a headache, a reverse engineer must thoroughly understand the platform he is dissecting to be successful in making a patch or deciphering a serial number.
To review: when a developer writes a program, he typically uses one of several languages. These include Visual Basic, C++, Java, or any one of the other, lesser-used languages. The choice of language depends on several factors; the most common are space and speed considerations. In the infamously bloated Windows environment, Visual Basic is arguably the king. This is because the hardware required to run Windows is usually more than enough to run any Visual Basic application. However, if a programmer needs a higher level of speed and power, he will probably select C++.
While these upper-level languages make programming easier by providing a large selection of Application Program Interfaces (APIs) and commands that are easy to understand, there are many occasions in which a programmer must create a program that can fit in a small amount of memory and operate quickly. To meet this goal, she may choose to use assembler, thus controlling the hardware of the computer directly. However, programming in assembler is tedious and must be done within an explicit set of rules.
Since every processor type uses its own set of assembler instructions, focus on one device (i.e., one processor type) and become fluent in the operation codes (opcodes), instruction sets, processor design, and methods by which the processor uses internal memory to read and write to RAM. Only after you master the basics of the processor operation can you start to reverse engineer a program. Fortunately, most processors operate similarly, with slight variations in syntax and use of internal processor memory.
Since our target in this chapter is the ARM processor used by PDAs, we provide some of the basic information you need to know, or at least to be familiar with, before attempting to study a program meant to run on this type of processor. The rest of this section describes the ARM processor, its major opcodes and their hex equivalents, and how its memory is used. If you do not understand this information, you may have some difficulty with the rest of this chapter.
The Advanced RISC Microprocessor (ARM) is a low-power, 32-bit microprocessor based on the Reduced Instruction Set Computer (RISC) principles. ARM is generally used in small devices that have a limited power source and a low threshold for heat, such as PDAs, telecommunication devices, and other miniature devices that require a relatively high level of computing power.
There are a total of 37 registers within this processor that hold values used in the execution of code. Six of these registers are used to store status values needed to hold the results of comparison and mathematical operations, among others. This leaves 31 registers to the use of the program, of which a maximum of 16 are generally available to the programmer. Of these 16, register 15 (R15) is used to hold the Program Counter (PC), which is used by the processor to keep track of where in the program it is currently executing. R14 is also used by the processor, as a subroutine link register (Lr), which is used to temporarily hold the value of R15 when a Branch and Link (BL) instruction is executed. Finally, R13, known as the Stack Pointer (Sp), is used by the processor to hold the memory address of the stack, which contains all the values about to be used by the processor in its execution.
In addition to these first 16 registers, some debuggers allow the programmer to monitor the last 4 registers (28-31), which are used to hold the results of arithmetic and logical operations performed by the processor (e.g., addition, subtraction, comparisons). Here’s a list of the registers and their purposes. They are listed in descending order because the processor bits are read from high to low.
- R31
Negative/less than
- R30
Zero
- R29
Carry/borrow/extend
- R28
Overflow
Understanding these registers is very important when debugging software. If you know what each of these values means, you should be able to determine the next step the program will make. In addition, using a good debugger, you can often alter these values on the fly, thus maintaining 100% control over how a program flows. Table 4-1 shows some possible conditional values and their meanings. It highlights the most common values that you will see in a debugger.
Table 4-1. Sample ARM conditional register values
Negative | Zero | Carry | Overflow | Meaning |
---|---|---|---|---|
0 | 0 | 0 | 0 | EQ—Z set (equal) |
0 | 0 | 0 | 1 | NE—Zero clear (not equal) |
0 | 0 | 1 | 0 | CS—Carry set (unsigned higher or same) |
0 | 0 | 1 | 1 | CC—Carry clear (unsigned lower) |
0 | 1 | 0 | 0 | MI—Negative set |
0 | 1 | 0 | 1 | PL—Negative clear |
0 | 1 | 1 | 0 | VS—Overflow set |
0 | 1 | 1 | 1 | VC—Overflow clear |
1 | 0 | 0 | 0 | HI—Carry set and Zero clear (unsigned hi) |
1 | 0 | 0 | 1 | LS—Carry clear and Zero set (unsigned lo or same) |
1 | 0 | 1 | 0 | GE—Negative set and Overflow set or Negative clear and Overflow clear (>=) |
1 | 0 | 1 | 1 | LT—Negative set and Overflow clear or Negative clear and Overflow set (<) |
1 | 1 | 0 | 0 | GT—Zero clear, and either Negative set and Overflow set or Negative clear and Overflow clear (>) |
1 | 1 | 0 | 1 | LE—Zero set, and either Negative set and Overflow clear or Negative clear and Overflow set (<=) |
1 | 1 | 1 | 0 | AL—Always |
1 | 1 | 1 | 1 | NV—Never |
Figure 4-1 illustrates Microsoft’s eMbedded Visual Tools (MVT) debugger, showing the values held in registers 0-12, Sp, Lr, and PC. In addition, this figure shows us the four registers (R31-R28) used to hold the conditional values. See if you can determine what condition the program is currently in, using Table 4-1.
Figure 4-1. MVT illustrating the registers
The ARM processor has a predefined set of operation codes (opcodes) that allows a programmer to write code. These same opcodes are used by compilers, such as Microsoft’s MVT, when a program is created for an ARM device. They are also used when a program is disassembled and/or debugged. For this reason, you must understand how opcodes are used, as well as what operations they perform. In addition, it is important to have a reference for the hex equivalent of each opcode, in order to find and replace an opcode as it appears in a hex dump of the file. While practice will ingrain the popular opcodes in your memory, this list will get you started.
The Branch opcode tells the processor to jump to another part of the program or, more specifically, the memory, where it will continue its execution. The B opcode is not to be confused with the Branch with Link (BL) opcode, discussed next. The main difference is that the B opcode is simply a code execution redirector. The program jumps to the specified address and continues processing the instructions. The BL opcode also redirects to another piece of code, but it eventually jumps back to the original code and continues executing where it left off.
There are several variations of the B opcode, most of which make obvious sense. The following is a list of the three most common variants and what they mean. Note that this list relates to the condition table in the previous section. In addition, we have included the hex code that you will need to search for when altering a Branch operation. For where to find a full list, please visit the Section 4.5 at the end of the chapter.
B Branch Always branches XX XX XX EA BEQ B if equal B if Z flag = 0 XX XX XX 0A BNE B if no equal B if Z flag = 1 XX XX XX 1AHere are some examples:
B loc_11498 07 00 00 EA BEQ loc_1147C 0C 00 00 0A BNE loc_11474 06 00 00 1AWhen a program is executing, there are situations in which the program must branch out and process a related piece of information before it can continue with the main program. This is made possible with a Branch with Link opcode. Unlike its relative, the B opcode, BL always returns to the code it was originally executing. To facilitate this, register 14 is used to hold the original address from which the BL was called.
The BL opcode has several variants to its base instruction, just like the B opcode. The following is a list of the same three variants and what they mean, which will be followed by examples. It is important to note that the examples show function calls instead of address locations. However, if you look at the actual code, you will find normal addresses, just like with the B opcode. The function naming convention is based on the fact that many BL calls are made to defined functions that return a value or perform a service. As you investigate CE reversing, you will become very intimate with the BL opcode. Note that the MVT debugger will not jump to the BL address when doing a line-by-line execution. It instead performs the function and continues to the next line. If you want to watch the code specified by the BL operation, specify a breakpoint at the memory address to which it branches. This concept is discussed later in this chapter.
BL Branch with Link Always branches XX XX XX EB BLEQ BL if equal BL if Z flag = 0 XX XX XX 0B BLNE BL if not equal BL if Z flag = 1 XX XX XX 1BHere are some examples:
BL AYGSHELL_34 7E 00 00 EB BLEQ mfcce300_699 5E 3E 00 0BA program is constantly moving data around. In order to facilitate this function, registers are updated with values from other registers and with hardcoded integers. These values are used by other operations to make decisions or perform calculations. This is the purpose of the Move opcode.
MOV does just what its name implies. In addition to basic moves, this opcode has the same conditional variants as the B and BL opcodes. By this point, you have a general understanding of what the EQ/NE/etc. means to an instruction set, so we will not discuss it further. Note, however, that almost every opcode includes some form of a conditional variant.
It’s important to understand how the MOV instruction works. This command can move the value of one register into another, or it can move a hardcoded value into a register. However, notice the item receiving the data is always a register. The following are several examples of the MOV command, what they do, and their hex equivalents.
MOV R2, #1 01 20 A0 E3 Moves the value 1 into R2 MOV R3, R1 01 30 A0 E1 Moves value in R1 into R3 MOV LR, PC 0F E0 A0 E1 Moves value of R15 into R14[1] MOV R1, R1 01 10 A0 E1 Moves value R1 into R1[2]Programs constantly need to compare two pieces of information. The results of the comparison are used in many ways: from the validation of a serial number, to continuation of a counting loop, etc. The assembler instruction set that is responsible for this process is Compare, or CMP.
The CMP operation can be used to compare the values in two registers with each other or to compare a register value and a hardcoded value. The results of the comparison do not output any data, but they do change the status of the conditional Zero flag. If the two values are equal, the Zero flag is set to 0; if the values are not equal, the flag is set to 1. This Zero value is then used by a subsequent opcode to control what is executed, or how.
The CMP operation is used in almost every serial number validation. The validation is accomplished in two ways: first, the actual comparison of the entered serial number with a hardcoded serial number; and second, after the validation check, when the program is deciding what piece of code is to be executed next. Typically, there will be a BEQ (Branch if Equal) or BNE (Branch if Not Equal) operation that uses the status of the Zero flag to either send a “Wrong Serial Number” message to the screen or accept the entered serial and allow access to the protected program. This use of the CMP operation is discussed further later in this chapter.
Another use of CMP is in a loop function. Loop functions assist in counting, string comparisons, file loads, and more. Being able to recognize a loop in a sequence of assembler programming is an important part of successful reverse engineering. The following is an example of how a loop looks when debugging a program.
00002AEC ADD R1, R4, R7 00002AF0 MOV R0, R6 00002AF4 BL sub_002EAC 00002AF8 ADD R5, R5, #20 00002AFC ADD R2, R5, #25 00002A00 CMP R3, R2 00002A04 BEQ loc_002AECThis is a simple loop included in an encryption scheme. In memory address 2A04, you can see a Branch occurs if the Zero flag is set. This flag is set, or unset, by memory address 2A00, which compares the values between R3 and R2. If they match, the code jumps back to memory address 2AEC.
The following are examples of two CMP opcodes and their corresponding hex values.
CMP R2, R3 03 00 52 E1 CMP R4, #1 01 00 54 E3While the registers are able to store small amounts of information, the processor must access the space allotted to it in the RAM in order to store larger chunks of information. This information includes screen titles, serial numbers, colors, settings, and more. In fact, almost everything that you see when you use a program has at one time resided in memory. The LDR and STR opcodes are used to write and read this information to and from memory.
While related, these two commands perform opposite actions. The Load (LDR) instruction loads data from memory into a register, and the Store (STR) instruction stores the data from the registry into memory for later usage. However, there is more to these instructions than the simple transfer of data. In addition to defining where the data is moved, the LDR/STR commands have variations that tell the processor how much data is to be moved. The following is a list of these variants and what they mean:
- LDR/STR
Move a word (four bytes) of data to or from memory.
- LDRB/STRB
Move a byte of data to or from memory.
- LDRH/STRH
Move two bytes of data to or from memory.
LDR/STR commands are different from the other previously discussed instructions in that they almost always include three pieces of information, due to the way the load and store instructions work. Since only a few bytes of data are moved, at most, the program must keep track of where it was last writing to or reading from. It must then append to or read from where it left off at the last read/write. You’ll often find LDR/STR commands in a loop where they read in or write out large amounts of data, one byte at a time.
The LDR/STR instructions are also different from other instructions in that they typically have three variables controlling where and what data is manipulated. The first variable is the data that is actually being transferred. The second and third variables determine where the data is written, and if it is manipulated before it is permanently stored or loaded. The following lists examples of how these instruction sets are used.
STR R1, [R4, R6] Store R1 in R4+R6 STR R1, [R4, R6]! Store R1 in R4+R6 and write the address in R4 STR R1, [R4], R6 Store R1 at R4 and write back R4+R6 to R4 STR R1, [R4, R6, LSL#2] Store R1 in R4+R6*2 (LSL discussed next) LDR R1, [R2, #12] Load R1 with value at R2+12. LDR R1, [R2, R4, R6] Load R1 with R2+R4+R6Notice the two new items that affect how the opcodes perform. The first is the “!” character, used to tell the instruction to write the new information back into one of the registers. The second is the use of the LSL command, which is discussed next.
Also related to these instructions are the LDM/STM instructions. These are also used to store or load register values; however, they do it on a larger scale. Instead of just moving one value, like LDR/STR, the LDM/STM instructions store or load all the register values. They are most commonly used when a BL occurs. When this happens, the program must be able to keep track of the original register values, which will be overwritten with values used by the BL code. So, they are stored into memory; then, when the branch code is completely executed, the original register values are loaded back into the registers from memory.
The above information should be easy to absorb for those of you who have previous experience with assembler or who are innately good programmers. However, if you are a newcomer, do not be discouraged, as mastering assembler typically takes years of dedicated study.
The final instruction sets we examine are the shifting operations. These are somewhat complicated, but they are a fundamental part of understanding assembler. They are used to manipulate data held by a register at the binary level. In short, they shift the bit values left or right (depending on the opcode), which changes the value held by the register. The following tables illustrate how this works with the two most common shifting instruction sets, Logical Shift Left, or LSL (Table 4-2), and Logical Shift Right, or LSR (Table 4-3). Because of space limitations, we will only be performing shifts on bits 0-7 of a 32-bit value. The missing bit values will be represented by ellipses (...).
Table 4-2. Logical Shift Left (LSL) shifts the 32-bit values left by x number of places, using zeros to fill in the empty spots
LSL | Original decimal | Original binary | New binary | New decimal |
---|---|---|---|---|
2 | 2 | ...00000010 | ...00001000 | 8 |
3 | 6 | ...00000110 | ...00110000 | 48 |
Table 4-3. Logical Shift Right (LSR) shifts the 32-bit values right by x number of places, using zeros to fill in the empty spots
LSR | Original decimal | Original binary | New binary | New decimal |
---|---|---|---|---|
4 | 30 | ...00011110 | ...00000001 | 1 |
3 | 25 | ...00011001 | ...00000011 | 3 |
While these are the most common shift instructions, there are three others that you may see. They are Arithmetic Shift Left (ASL), Arithmetic Shift Right (ASR), and Rotate Right Extended (ROR). All of these shift operations perform the same basic function as LSL/LSR, with some variations. For example, the ASL/ASR shifts fill in the empty bit places with the bit value of register 31, which preserves the sign bit of the value being held in the register. The ROR shift, on the other hand, carries the bit value around from bit 0 to bit 31.
The previous pages have given you a brief look at assembler programming on ARM processors. You will need this information later in this chapter when we practice some of our RCE skills on a test program—it will be valuable as you attempt to debug software, find exploits, and dissect hostile code.
For this section, you will need to use the tools described in previous chapters, including hex editors and disassemblers. We start by creating a simple “Hello World!” application, and we then use this program to demonstrate several cracking methods. After this discussion, we offer a hands-on tutorial that allows you to walk through real-life examples of how reverse engineering can be used to get to the heart of a program.
When learning a programming language, the first thing most people do is to create the famous “Hello, World” application. This program is simple, but it helps to get a new programmer familiar with the syntax structure, compiling steps, and general layout of the tool used to create the program. In fact, Microsoft’s eMbedded Visual C++ goes so far as to provide its users with a wizard that creates a basic “Hello World” application with the click of a few buttons. The following are the required steps:
Open Microsoft eMbedded Visual C++.
Click File → New.
Select the Projects tab.
In the “Project Name:” field, type “test”, as illustrated in Figure 4-2. Select WCE Application on the left.
Figure 4-2. WCE application creation window
Tip
By default, all compiled executables will be created in the C:\Program Files\Microsoft eMbedded Tools\Common\EVC\MyProjects\ directory.
Click OK.
Ensure “A typical `Hello World!’ Application” is selected, and click Finish.
Click OK.
Tip
We’re running the programs on a PDA synchronized with our computer, but the beauty of Microsoft’s eMbedded Visual Tools is you don’t need a real device. The free MVT has an emulator for virtual testing .
After a few seconds, a new “test” class appears on the left side of the screen, under which are all the classes and functions automatically created by the wizard. We aren’t making any changes to the code, so next, we compile and build the executable:
Ensure the device is connected via ActiveSync.
Click Build →test.exe.
Click Yes/OK through the warnings.
Locate the newly created executable in your C:\Program Files\Microsoft eMbedded Tools\Common\EVC\MyProjects\ directory, or whatever directory you selected during the wizard, and copy it to your device.
Once the steps are complete, find test.exe on your device and execute it. If everything went according to plan, you’ll see a screen similar to Figure 4-3. After a short break to discuss some of the popular methods crackers use to subvert protection, we will take a closer look at test.exe and make some changes to it using our reversing tools.
Figure 4-3. test.exe screen on the Windows CE device
In this section, we briefly review some of the cracking techniques discussed in earlier chapters and apply them to embedded reverse engineering. Users who feel comfortable with the Windows CE OS can skip to Section 4.3.3.
In about 80% of all software, there is a common flaw that leads to the eventual cracking of the software: predictable code. For example, if you go through the registration process, you will almost always find a message that tells you the wrong serial number was entered. While this is a nice gesture for the honest person who made a mistake, it is a telltale sign that the program is an easy crack.
The problem arises simply because there are a limited number of alert boxes that appear in a program. A cracker has only to open the program in IDA Pro and search the strings for any calls made to MessageBoxW—the name of the function responsible for sending a message to the computer screen.
Once the cracker finds this call, she can use the reference list included with IDA Pro to backtrack through the program until she finds the point where the serial number is verified. In other words, using a message box to warn about an invalid serial gives the cracker the necessary starting point to look for a weakness. Without it, a beginner cracker could spend hours slowly stepping through the program, testing and probing.
Other common calls are Load String (for loading serial number values into a variable), Registry checks (for checking to see if the program is registered or not), and System Time checks (for checking for trial period deadlines). To find these, a cracker only has to use the Names window, which lists all the functions and system calls used in the program. Figure 4-4 is taken from IDA Pro, with our test.exe program loaded into it. The highlighted function may be a good place to start when looking for a way to alter the displayed message.
Figure 4-4. Names window in IDA, listing the CE functions used
When working with strings such as usernames, serials, or other text entries, it is important to monitor the length. The length of the string is important for two reasons. One, a program that expects a string may generate an error if it receives a variable with no value. For example, if a program is trying to divide two numbers and the denominator is blank, the calculation will fail. To avoid problems like this, a program will include checks to ensure that a value is indeed entered.
The second main use of string length checks is when setting aside memory for a variable. For example, our “Hello, World!” application must set aside enough memory for a 12-character variable. The program checks to see how much space is required using wcslen, as the following code illustrates:
ADD R0, SP, #0x54; Points R0 to memory address of 'Hello World!' string. BL wcslen; Tests the length of the string and places that value in R0.While testing string length is undeniably important, it is also an easy function to find and abuse. Because these types of functions are required when verifying serial numbers, a cracker has only to look in the Names window of the application to start the reversing process. In fact, crackers sometimes target this check and reset the required serial number length to zero, thus bypassing a program’s security.
Another popular method of finding serial number checks is through the use of the comparison (CMP) instruction. This type of function is used to compare two values to see if they are equal, and it can flip the Zero flag to true or false accordingly. Again, this is a required function for program execution; however, it comes with a serious risk.
Using strcmp or CMP as the sole method of validation in a registration process is not recommended. This particular function is one of the most abused and exploited functions in assembler. In fact, the use of this one little command can sometimes neuter a program that uses complex serial verification routines with encryption, name checks, and more.
For example, some programs do not actually store their serial numbers in the program file. Instead, an algorithm is used to create a valid serial number on the fly, based on owner names, hardware settings, the date/time, and more. In other words, thousands of lines of code are dedicated to creating a valid registration key. This key is used in the validation process to check any serial number that is entered to unlock a program. However, at the very end of the verification routine, most programs simply perform a simple comparison between the entered serial number and the one generated by the complex algorithm. The results of this check are placed into one of the registers, which are used to determine how the program flows. Typically, the next line includes some conditional branch call that either accepts the entered serial number or rejects it. Let’s take a look at the following example, in which strcmp is used to verify a registration value:
Assume R1 = address of correct serial ADD R0, SP, #0x12 : This updates RO with a value pulled from the stack, which corresponds to the serial : number entered by the user. BL strcmp : This compares the values held in addresses that R0 and R1 point to and sets the : Zero flag accordingly: 1 for no match and 0 for match. MOVS R2, R0 : Writes the value of R0 into R2 (the entered serial number). MOV R0, #0 : Assigns R0 = 0 CMP R2, R0 : The CMP will check R0 against the value held by R2 (the results of the strcmp); : if these values match, then the serials do not match.Following this function, there would be a branch link to another section of code that would update the serial status and probably alert the user to a success or failure of the registration attempt. This would be done using the status flags, updated when the CMP opcode was executed. The following is an example:
BNE loc_0011345 BEQ loc_0011578Therefore, if a cracker wanted to patch this program, he would only need to ensure that the CMP opcode always worked to his advantage. To do this, he would update the following opcode:
CMP R2, R1 CMP R2, R2Since R2 will always equal R2, the CMP updates the status flags with an Equal status. This is used in the BNE/BEQ branches, which react with a positive serial check. To do this, a cracker would have to update the hex values as follows:
CMP R2, R1 Hex: 01 0 52 E1 CMP R2, R2 Hex: 02 0 52 E1In other words, thanks to strcmp and the change of one hex character, the protection of this program is nullified.
When attacking a program, there are some situations that require a cracker to overwrite existing code with something known as a nonoperation (NOP). A nonoperation simply tells the processor to move on to the next command. When a series of NOP commands are used in sequence, the processor virtually slides through the code until it hits a command it can perform. This technique is popular in both the hacking and cracking community, but for different reasons.
A hacker typically uses NOP slides to facilitate the execution of inserted code through a buffer overflow. A buffer overflow (discussed in Chapter 5) is a method of overflowing a variable’s intended memory allocation with data. This allows a hacker to write her own code right into the memory, which can be used to create a backdoor, elevate permissions, and more. However, a hacker does not always know where her code ends up in the target computer’s memory, so she typically pads her exploit code with NOP commands. This allows a hacker to guess where in the memory to point the execution code. Upon hitting the NOP commands, the processor just slides into the exploit code and executes it.
A cracker, on the other hand, does not use NOP slides to execute code. Instead, he uses NOP commands to overwrite code he does not want executed. For example, many programs include a jump or branch in the assembler code that instructs the processor to validate a serial number. If a cracker can locate this jump in the program, he can overwrite it with a NOP command. This ensures that the program remains the same byte size and bypasses the registration check. Typically, this method will also be used with a slight alteration on a compare or equivalence function, to ensure proper continued code execution.
Traditionally, the NOP command is as simple as typing 0x90 over the hex that needs to be nullified. However, this works only on an x86 processor, not on ARM. If you attempt to use 0x90s on ARM, you end up inserting UMULLSS, which is the command to perform an unsigned multiply long if the LS condition flags are set, followed by an update of the status flags depending on the result of the calculation. Obviously, this is about as far from a NOP as you can get.
Ironically, the ARM processor has no true NOP command. Instead, a cracker would need to use a series of commands that essentially perform no operation. This is accomplished by simply moving a value from a register back into itself, as follows:
(MOV R1, R1)This method of cracking is common because it is one of the easiest to implement. For example, if a cracker wanted to bypass a “sleep” function in a shareware program, she could easily search for and find something similar to the following code.
Assembler HEX MOV R0, #0x15 15 00 A0 E3 BL Sleep FF 39 00 EB MOV R4, R0 00 40 A0 E1Using a hex editor, a cracker would only have to make the following changes to the code to cause the “sleep” function to be ignored:
Assembler HEX MOV R0, #0x15 15 00 A0 E3 MOV R1,R1 MOV R4, R0 00 40 A0 E1Note the missing command. When you overwrite this command, the revised program will not display, for example, a nag screen that temporarily restricts access. Instead, the user will be taken straight into the program.
To our knowledge, at the time of this writing there are no hex editors that work directly on Windows Mobile platforms. However, you can edit the application on the desktop (Figure 4-5) using methods described in previous chapters.
Figure 4-5. UltraEdit-32 hex output of test.exe
Disassembling a CE Program
As discussed previously, a disassembler is a program that interprets machine code into a language that humans can understand. Recall that a disassembler attempts to convert hex/binary into its assembler equivalent. However, there are as many different assembler languages as there are types of processors. AMD, Intel, and RISC processors each have their own languages. In fact, processor upgrades often include changes to the assembler language, to provide greater functionality.
As a result of the many variations between languages, disassembling a program can be challenging. For example, Microsoft’s MVT, discussed next, includes a disassembler to allow for CE debugging. However, this program will not debug code meant to run on a Motorola cell phone. This is why choosing the right debugger is an important process—which brings us to IDA Pro.
Once you have obtained a copy of IDA Pro, execute it and select New from the pop-up screen. You will be prompted for a program to disassemble. For this exercise, we will use the test.exe file that we just created. However, we are going to alter the file and control the execution of the program to show a different message than the one it was originally programmed for.
The first thing you need to do is load the test.exe file into IDA Pro. You need to have a local copy of the file on your computer. Step through the following instructions to get the test.exe file disassembled.
Open IDA (click OK through splash screen).
Click New at the Welcome screen and select test.exe from the hard drive; then, click Open.
Check the “Load resources” box, change the “Processor type” drop-down menu selection to “ARM processors: ARM,” and click OK, as illustrated in Figure 4-6.
Click OK again if prompted to change the processor type.
Tip
At this point you may be asked for some *.dll files. We recommend that you find the requested files (either from MVT or from your device) and transfer them to a local folder on your PC. This allows IDA to fully disassemble the program. test.exe requires the AYGSHELL.DLL file, which can be downloaded from the Internet.
Locate any requested *.dll files and wait for IDA to disassemble the program.
If the Names window does not open, select it from the View → Open Subviews → Names menu.
Locate “LoadStringW” from the list and double-click on it.
Figure 4-6. IDA Pro startup configuration for test.exe
At this point, you should have the following chunk of code listed at the top of the disassembler window:
.text:00011564 ; S U B R O U T I N E .text:00011564 .text:00011564 .text:00011564 LoadStringW ; CODE XREF: sub_110E8+28#p .text:00011564 ; sub_110E8+40#p ... .text:00011564 LDR R12, =_ _imp_LoadStringW .text:00011568 LDR PC, [R12] .text:00011568 ; End of function LoadStringWIf you look at this code, you can see that LoadStringW is considered a subroutine. A subroutine is a mini-program that performs some action for the main program. In this case, it is loading a string. However, you will want to pay attention to the references that use this subroutine. These will be listed at the top of the routine under the CODE XREF, which stands for cross-reference. In our case, there are two addresses in this program that call this subroutine; they are sub_110E8+28 and sub_110E8+40. While these addresses may appear a bit cryptic, they are easy to understand. In short, the cross-reference sub_110E8+28 tells you that this LoadStringW subroutine was called by another subroutine that is located at address 110E8 in the program. The actual call to LoadStringW was made at the base 110E8 address plus 28 (hex) bytes of memory into the routine.
Tip
Not all XREFs are always visible. If there are more than two, there will be a “...” after the second reference.
While it is possible to scroll up to this memory location, IDA makes it easy by allowing us to click on the reference. Here’s the secret: right-click on the “...” and select the “Jump to cross reference” option. Select the third option on the list, which should be 1135C. Without this shortcut, you would have to go to each XREF and check to see where in the display process the code is.
Once at address 1135C, you can see that it looks very promising. Within a short chunk of code, you have several function calls that seem to be part of writing a message to a screen (i.e., BeginPaint, GetClientRect, LoadStringW, wcslen, DrawTextW). Now we will use the lessons we’ve learned to see what we can do.
As we learned, wcslen is a common point of weakness. We are going to use this knowledge to change the size of our message. Let’s take a closer look at this part of the code, assuming that the message is loaded into memory.
.text:0001135C BL LoadStringW ;load string .text:00011360 ADD R0, SP, #0x54 ;change value of ;R0 to point to string location .text:00011364 BL wcslen ;get length of ;string and put value in R0 .text:00011368 MOV R3, #0x25 ;R3 = 0x25 .text:0001136C MOV R2, R0 ;moves our string ;length into R2 .text:00011370 STR R3, [SP] ;pushes R3 value ;on memory stack .text:00011374 ADD R3, SP, #4 ;R3 = memory stack ;address + 4 .text:00011378 ADD R1, SP, #0x54 ;R1 = memory stack ;address + 0x54 .text:0001137C MOV R0, R5 ;moves R5 to R0 .text:00011380 BL DrawTextW ;writes text to ;screen using R0, R1, R2 to define ;location of string in memory, ;length of string, and type of draw.Now that we have broken down this part of the code (which you will be able to do with practice), how can we change the length of the string that is drawn to the screen? Since we know that this value was moved into R2, we can assume that R2 is used by the DrawTextW routine to define the length. In other words, if we can control the value in R2, we can control the message on the screen.
To do this, we only need to change the assembler at address 1136C. Since R2 gets its value from R0, we can simply replace the R0 variable with a hardcoded value of our own. Now that we know this, let us edit the program using our hex editor.
Once you get the hex editor open, you will quickly see that the address in IDA does not match the address in the hex editor. However, IDA does provide the address in another part of the screen, as illustrated in Figure 4-7. The status bar located at the bottom left corner of the IDA window gives the actual memory location you need to edit.
Figure 4-7. IDA Pro status bar showing memory address
Using the opcodes discussed previously in this chapter, you recreate the hex code you want to use in place of the existing code. The following is the original hex code and the code you will want to replace it with.
Here is the original:
MOV R2, R0 00 20 00 E1And here it is, updated:
MOV R2, 1 01 20 00 E3Note the change from E1 to E3; it differentiates between a MOV of a register value and a MOV of a hardcoded value.
What did this change accomplish? If you download the newest test.exe file to your PDA, you will see that it now has a message of just “H”. In other words, we caused the program to only load the first character of the message it had stored in memory. Now, imagine what we could do if we increased the size of the message to something greater than the message in memory. Using this type of trick, a cracker could perform all kinds of manipulation. However, these types of tricks often take more than just a disassembler, which is where MVT comes in handy.
Microsoft’s eMbedded Visual Tools
Currently, there are very few tools available for live debugging of Windows CE devices. The choice of free tools is even more limited. However, Microsoft, in its benevolent wisdom, has provided just such a tool. You will need this tool to reverse engineer most Windows CE applications, unless you are intimately familiar with ARM assembler. Even if you do know the ARM code, the debugger will allow you to access parts of a program that you cannot access via a disassembler.
In short, MVT allows you to run a program, one line or opcode at a time. In addition, it allows you to observe the memory stack, register values, and values of variables in the program while it is executing. And if that isn’t enough, the debugger allows you to actually change the values of the registers and variables while the program is executing. With this power, you can change a Zero flag from a 1 to a 0 in order to bypass a protection check, or even watch the program compare an entered serial number with the hardcoded number, one character at a time. Needless to say, a debugger gives you total control over the program. It not only lets you look at the heart of its operation, but allows you to redesign a program on the fly.
To illustrate this power, we will use our little example program again. We will change the message on the screen, but this time we will locate the hardcoded message in memory and redirect the LDR opcode to a different point in the memory. This has the effect of allowing us to write whatever message we want to the screen, providing it exists in memory.
The first step in debugging a program is to load it into the MVT. This step typically involves the use of the Microsoft eMbedded Visual C++ (MVC) program that is included with the MVT package. Once C++ is open, perform the following steps to load the test.exe file into your debugger. Optionally, if you have a Windows Mobile device, you will want Microsoft ActiveSync loaded, with the device connected. In this case, be sure to have a copy of the test.exe file stored on the CE device, preferably under the root folder.
Open Microsoft eMbedded Visual C++.
Select File → Open.
Change “Files of type:” to “Executable Files” (.exe, .dll, .ocx).
Select the local copy of test.exe.
After brief delay, select Project → Settings from the top menu.
Click the Debug tab.
In the “Download directory:” text box, type “\” (or point the directory to the folder you have selected on the CE device).
Click OK, and then hit F11.
You will see a Connecting screen (Figure 4-8) followed by a warning screen (Figure 4-9). Select Yes on the CPU Mismatch Warning dialog window.
Figure 4-8. Microsoft eMbedded Visual C++ connecting screen
Figure 4-9. Microsoft eMbedded Visual C++ CPU warning
Figure 4-10. Microsoft eMbedded Visual C++ platform warning
The file will download and some file verification will occur.
Click OK on the debugging information warning screen (Figure 4-11).
Automobile License Plate Collectors Association
![]() | |
Membership driven | |
Industry | History, Research |
---|---|
Founded | December 22, 1954; 65 years ago (December 22, 1954) in Rumney Depot, New Hampshire, U.S., incorporated December 1957 |
Founders | Dr. Cecil George, Asa P. Colby |
Headquarters | |
Worldwide | |
Cyndi McCabe (President and Chairman of the Board) | |
Products | Memberships, magazine, archives, conventions, annual Best Plate Award |
Website | https://www.alpca.org |
The Automobile License Plate Collectors Association (ALPCA) is the largest such organization in the world.[1] Founded in Rumney Depot, New Hampshire, United States, in 1954 and holding its first meeting/convention in North Attleboro, Massachusetts, in 1955 it serves as a way for license plate collectors to trade plates, distribute news, and provide historic information to members.[2]
Overview[edit]

ALPCA currently has approximately 2,800 registered members and over 13,100 registered member numbers,[1] although many of the earlier—and therefore lower-numbered—members are now deceased or are no longer members. When an individual joins, he or she is assigned a membership number, which started with #1 in 1954, and are currently being issued in the 13,200 range. Members treasure their "ALPCA number" and often are known as much by the time period in which they joined than by name or geographic home. The club hosts an annual convention each June or July that attracts several hundred members, and regional meets take place in a number of states, provinces, and countries throughout the year. Due to the COVID 19 pandemic the 2020 convention, originally scheduled for Tulsa, Oklahoma from July 8-11, 2020, was cancelled. The next convention, open to members only, will be in Covington, Kentucky, from June 23 to 26, 2021.[3][4]
Members come from all 50 U.S. states and the District of Columbia, as well as nineteen countries. Most members reside in the United States, followed by Canada, Germany, and Australia. Members receive the bi-monthly full-color publication "Plates;" have full access to the organization's web-based license plate archives for every country around the world; access to back issues of all publications; and can attend both regional meets and the annual convention.[5]
Despite the club's name, many members also collect and exchange license plates from vehicles other than automobiles. Antique vehicle, bus, camper, farm, military, motorcycle, trailer, and truck plates are just a few of the hundreds of other plate types that have been issued and are now collected by members.[6][7]
Since 1972 ALPCA has annually presented a "Best Plate Award" to an American state or Canadian province.[8] To better educate the public regarding genuine license plates the association provides a "Guide to Deceptive and Misleading Plates" for free on its website.[9]
Many ALPCA members maintain their own interests and websites, sometimes overlapping, and sometimes going way beyond the confines of license plate collectors. A subset of them are "Extra-Miler Club" members, who seek to visit every county in the United States, often while picking antique shops and other venue for old tags. One member keeps track of the "highest numbers" issued in each state, and his website records what combinations are the latest to be issued.[10]
ALPCA members often consult the media for stories on issues in automobilia.[11][12][13]
Membership[edit]
The ALPCA has approximately 2,800 current members. Members choose whether their membership lasts one, two, three, four, or five years. Junior memberships, for those members less than 18 years of age, are also available. The organization's magazine, "Plates," is mailed to all members, and all members in good standing are entitled to vote for officers and the Best Plate Award. Members can research plates with the association's online archives which have been extensively expanded and updated in recent years. Members may also display the ALPCA logo (shield) for personal use.[14]
Executive Board[edit]
The ALPCA is run by a seven member Executive Board composed of the President, Vice-President, and five Directors. All members serve two year terms. The President and three of the Directors terms expire in even numbered years, and the Vice-President and two Directors terms expire in odd numbered years. The Administrative Staff, composed of the Secretary, Treasurer, Plates Editor, Webmaster, Regional Meet Coordinator, Complaint Chairman, and Legal Counsel, are appointed by the Executive Board, Similarly, the Project Staff, consisting of the Convention Planner, Convention Administrator, Archivist, Hall of Fame Coordinator, and Best Plate Coordinator, are also appointed by the Executive Board. The President also serves as the Chairman of the Executive Board. Note that prior to the 1977 election of board members there were multiple other elected offices which are not shown here.[15][16]
Position | Name | Location | Term Ending |
---|---|---|---|
President | Cyndi McCable | Elyria, OH | 7/7/2020 |
Vice-President | Dick Yourga | Amherst, MA | 6/22/2021 |
Director | Greg Gibson | Fenton, MI | 7/7/2020 |
Director | Bill Dickerson | Manchester, NH | 6/22/2021 |
Director | Joel Kimmet | Cincinnati, OH | 6/22/2021 |
Director | Andrew Pang | Arlington, VA | 7/7/2020 |
Director | Stephen Tuday | Woodstock, GA | 7/7/2020 |
List of ALPCA Presidents[edit]
The ALPCA presidents' term of office has changed two times. From 1955 until 1961 their term was two years, from 1961 until 2004 the term was one year, and since 2004 the term is once again two years. To simplify the following chart multiple terms served consecutively are combined in one row. The following list of ALPCA Presidents was compiled from the ALPCA Newsletter, ALPCA Register, and Plates Magazine.[15]
No. | Name | Dates | Terms | Notes |
---|---|---|---|---|
1 | Dr. Cecil W. George | 1955–1957 | 1 | Member #1; Granted life membership in 1988[17] |
2 | Ralph Perkins | 1957 – July 4, 1958 | 1 | Resigned |
3 | Frank Spinney | July 5, 1958 – 1959 | 1 | Appointed |
4 | Roy A. Carson | 1959–1961 | 1 | |
5 | Asa P. Colby | 1961–1962 | 1 | |
6 | Clarence Titus | 1962–1963 | 1 | |
7 | Carl Sweiven | 1963–1964 | 1 | |
8 | Harvey Parkes | 1964–1966 | 2 | |
9 | Andrew Young | 1966–1967 | 1 | |
10 | Ernie Wilson | 1967–1968 | 1 | |
11 | Joe Oliver | 1968–1969 | 1 | |
12 | Frank German | 1969–1972 | 3 | |
13 | Paul M. Maginnity | 1972–1973 | 1 | |
14 | Willie Stahl | 1973–1974 | 1 | |
15 | Earl Jenson | 1974–1975 | 1 | |
16 | Edward Dietz | 1975–1976 | 1 | |
17 | Dick Pack | 1976–1977 | 1 | |
18 | Arthur D. Currie | 1977–1979 | 2 | |
19 | James A. Crilly | 1979–1981 | 2 | |
20 | Robert M. Crisler | 1981–1983 | 2 | |
21 | James K. Fox | 1983–1985 | 2 | |
22 | Robert M. Crisler | 1985–1987 | 2 | |
23 | Jeffrey C. Minard | 1987–1989 | 2 | |
24 | James A. Crilly | 1989–1991 | 2 | |
25 | Michael C. Wiener | 1991–1993 | 2 | |
26 | James K. Fox | 1993–1995 | 2 | |
27 | Dale W. Blewett | 1995–1997 | 2 | |
28 | Richard Yourga | 1997–2000 | 3 | |
29 | Barney Schukraft | 2000–2001 | 1 | |
30 | Michael R. Naughton | 2001–2006 | 4 | Three single year terms and one two year term. |
31 | Jeff Francis | 2006–2010 | 2 | |
32 | Michael Liscio | 2010 – May 30, 2011 | 1 | Resigned |
33 | Stephen Tuday | June 1, 2011 – July 2011 | 1 | Resigned |
34 | Greg Gibson | July 2011 – 2016 | 3 | |
35 | Cyndi McCabe | 2016 – | 2 |
Events[edit]
International conventions[edit]
The ALPCA has held annual conventions since 1955. The four earliest conventions were held at the host members' home. Since 1959 the annual conventions have been held in a larger private or public venue, and those held since 1984 have been at a convention center or other similarly large public venue. Conventions include three days of selling and trading of plates on the convention floor, an auction of license plates donated by members and individual states' department of motor vehicles, member displays that are judged and presented awards in many categories, committee and membership meetings, and social activities. Newly elected Executive Board members are installed just prior to the convention start. Conventions are only open to members and their guests.[18]
No. | Year | Venue | Location |
---|---|---|---|
1 | 1955 | Property of Cecil George | No. Attleboro, MA[18] |
2 | 1956 | Property of Cecil George | No. Attleboro, MA[18] |
3 | 1957 | Property of Robert Oliver | Setauket, NY |
4 | 1958 | Property of Frank Spinney | Bristol, NH[19] |
5 | 1959 | Yorklyn Gun Club | Yorklyn, DE |
6 | 1960 | Odd Fellows Hall | Adamsburg, PA |
7 | 1961 | Giroux Motor Company | Phillipsburg, NJ[20] |
8 | 1962 | American Legion Hall | North East, MD |
9 | 1963 | American Legion Hall | Canastota, NY |
10 | 1964 | Grange Hall, Hancock County Fair Grounds | Findlay, OH[21][22] |
11 | 1965 | Natural Bridge Hotel | Natural Bridge, VA[23][24] |
12 | 1966 | Campus Center, Otterbein College | Westerville, OH[24] |
13 | 1967 | Franklin County Fair Grounds | Greenfield, MA[25] |
14 | 1968 | Merchants Building, Hancock County Fair Grounds | Findlay, OH[26] |
15 | 1969 | Memorial Gymnasium, Juniata College | Huntingdon, PA[27] |
16 | 1970 | Kane County Fairgrounds | St. Charles, IL |
17 | 1971 | Youth Center, Ohio State Fairgrounds | Columbus, OH |
18 | 1972 | Utah National Guard Armory | Salt Lake City, UT |
19 | 1973 | Lakeside Gardens, Britannia Park | Ottawa, ONT, CAN[28] |
20 | 1974 | Hoover Community Building, Garfield Fair Grounds | Enid, OK[29] |
21 | 1975 | Acadia Community Hall | Calgary, ALB, CA[30] |
22 | 1976 | Juniata College | Huntingdon, PA |
23 | 1977 | Salem Community High School | Salem, IL |
24 | 1978 | Lehigh Resort Motel | Lehigh Acres, FL |
25 | 1979 | Lakeland Community College | Mentor, OH |
26 | 1980 | Oklahoma State Fairgounds | Oklahoma City, OK[31] |
27 | 1981 | Central Cabarrus High School | Concord, NC |
28 | 1982 | Lake Michigan College | Benton Harbor, MI |
29 | 1983 | Dulaney Senior High School | Timonium, MD[32] |
30 | 1984 | Rushmore Plaza Civic Center | Rapid City, SD |
31 | 1985 | Asheville Civic Center | Ashville, NC[33] |
32 | 1986 | Springfield Convention Center | Springfield, IL[33] |
33 | 1987 | Abuquerque Convention Center | Albuquerque, NM |
34 | 1988 | Arthur Ashe Center | Richmond, VA |
35 | 1989 | Riverside Convention Center | Rochester, NY |
36 | 1990 | Rapid City Convention Center | Rapid City, SD |
37 | 1991 | Kansas City Market Center | Kansas City, MO |
38 | 1992 | Peoria Civic Center | Peoria, IL |
39 | 1993 | Hammons Trade Center, Aurora | Denver, CO[34] |
40 | 1994 | Chattanooga/Hamilton County Conv. & Trade Ctr. | Chattanooga, TN |
41 | 1995 | Sea Gate Convention Centre | Toledo, OH |
42 | 1996 | Peoria Civic Center | Peoria, IL |
43 | 1997 | Tucson Convention Center | Tucson, AZ |
44 | 1998 | Niagara Falls Convention and Civic Center | Niagara Falls, NY |
45 | 1999 | Chattanooga/Hamilton County Conv. & Trade Ctr. | Chattanooga, TN |
46 | 2000 | Hammons Trade Center, Aurora | Denver, CO |
47 | 2001 | Peoria Civic Center | Peoria, IL |
48 | 2002 | Niagara Falls Convention and Civic Center | Niagara Falls, NY |
49 | 2003 | Tucson Convention Center | Tucson, AZ |
50 | 2004 | Rhode Island Convention Center | Providence, RI |
51 | 2005 | Sioux Falls Convention Center | Sioux Falls, SD |
52 | 2006 | Ontario Convention Center | Ontario, CA |
53 | 2007 | Von Braun Center | Huntsville, AL |
54 | 2008 | Salt Palace Convention Center | Salt Lake City, UT |
55 | 2009 | Bayfront Convention Center | Erie, PA |
56 | 2010 | Arlington Convention Center | Arlington, TX |
57 | 2011 | Charleston Convention Center | Charleston, WV[35] |
58 | 2012 | Iowa Events Center | Des Moines, IA |
59 | 2013 | Grand Sierra Resort | Reno, NV |
60 | 2014 | Rochester Riverside Convention Center | Rochester, NY |
61 | 2015 | Northwest Arkansas Embassy Suites | Rogers, AR |
62 | 2016 | Grand Wayne Convention Center | Fort Wayne, IN[36] |
63 | 2017 | Ontario Convention Center | Ontario, CA |
64 | 2018 | Valley Forge Casino Resort | Valley Forge, PA |
65 | 2019 | Knoxville Convention Center | Knoxville, TN |
---- | 2020 | Cox Business Center | Tulsa, OK (cancelled due to COVID-19 pandemic)[37] |
66 | 2021 | Northern Kentucky Convention Center | Covington, Kentucky[38] |
Regional meets[edit]
Regional meets provide the opportunity for members to sell, trade, or barter plates with other members between annual conventions. The dates for officially sanctioned meets are published in the organization's magazine "Plates." These meets often include auctions, regional meetings, awards, and a social event such as a lunch meal. Like the annual conventions, regional meets are only open to members and invited guests. The number of regional meets varies each year, but recent years have seen 15 – 25 regional meetings. Some regional groups hold a single meeting, while others may host quarterly meetings. A list of upcoming regional meetings is also maintained at the ALPCA website.[39][40]
Independent meets[edit]
Although these meets are not sponsored by ALPCA they provide some of the same collecting opportunities that the ALPCA annual convention and officially sanctioned regional meets provide. The independent meets also allow the general public a way to meet ALPCA members, help members learn about plates by talking to sellers, and begin or add to their collections of license plates. A list of upcoming independent meetings is maintained at the ALPCA website.[41]
Publications[edit]
From its inception ALPCA has distributed a publication to all active members. Unofficially, the primary publication was titled the ALPCA Newsletter, but it was many years before this was formalized. The name first appeared on the masthead in April 1972, but the name had appeared in the newsletter itself many times before this date. The ALPCA Newsletter changed names in February 2000 to the ALPCA Register, and since December 2004 has been known as Plates Magazine or informally as Plates. Back issues of these publications are available to active members on the association's website.[42][43][44]
Plates Magazine[edit]
Plates Magazine is full color and regularly features articles relating the history of a topic, such as an individual state, province, or country's plates; provides information about newly issued plate; convention, regional, and independent meet details; photo histories of plates and related ephemera, such as validation stickers or tabs; topical histories such as motorcycle, special event, ham radio, personalized plates; articles on individual collectors or collections; annual base plate information for North American jurisdictions; etc.[45][46][47][48]
Archives[edit]
The online archives provide members an extensive research tool for information regarding the year a license plate was issued, serial numbers issued, colors, dates issued, slogans, plate material, and other plate characteristics. Originally relatively static, the archives have been greatly expanded, become an online database that is much easier to search, sort, and provides much improved photos over the original archives.[49]
Book[edit]
For the 50th anniversary of ALPCA two members wrote a book detailing the associations history. This publication, "A Moving History, 50 Years Of ALPCA, 100 Years of License Plates" was written by Jeff Minard & Tim Stentiford, and published by Turner Communications. In addition to the ALPCA history the book details 100 years of license plate history, and over 275 profiles of a cross section of ALPCA members.[50][51]
Best Plate Award[edit]
Since 1972 members have voted each year to name one United States or Canadian license plate design, and in some years two such designs, introduced during the previous year as the "Best Plate."[52] The first award went to Pennsylvania for their early Bicentennial plate which featured the Liberty Bell in the center.[52] The award to the 1970 Northwest Territories for their polar bear-shaped license plate was made by Executive Board action between December 1973 and February 1974.[53]
Members votes are based on both the plate's legibility and its aesthetics. For 1995, 1997, and 1998, the club recognized one standard-issue plate and one optional-issue plate, while in 1985 and 1989, two jurisdictions' designs tied for first place.[8] The presentation of the award each year is usually covered by media outlets in the state that wins the award.[54][55]
Hall of Fame[edit]
The ALPCA Hall of Fame was approved by the Board of Directors in 2002, but it did not officially induct any members until 2004. "The goal of the Hall of Fame is [to] provide a vehicle to learn about our founding fathers and the legacy of leaders throughout the years who have embodied the fraternal spirit of our hobby."[80]
"To be considered for the Hall of Fame, candidates must have served the club or hobby unselfishly for the good of all without concern for praise, compensation, or reward; promoted membership; provided guidance to fellow members; and taught others the value of collecting."[80] Any member in good standing may nominate any ALPCA member for induction into the hall that they feel is deserving of the honor. The Hall of Fame Committee reviewes all initial nominations, and the past presidents of the association vote to determine who on the list would be inducted in the Hall of Fame.[80]
|
|
|
|
Notable members[edit]
- Robert M. "Bob" Crisler, of Lafayette, Louisiana, served as an officer of ALPCA and was inducted as one of the first members of its Hall of Fame. He is a retired geography professor from the University of Louisiana, and authored "License Plate Values," the first collector price guide in the hobby.[81]
- Jim Fox, drummer of the James Gang; served as an officer of the ALPCA and authored one of the most prominent published works within the hobby, License Plates of the United States.
- Ernest R. Hemmings, of Quincy, Illinois, the founder of Hemmings Motor News.
- Keith Marvin, of Menands, New York, wrote three books about license plates and seven books total. He wrote over 3,000 articles on automotive history, and his work appeared in more than 70 different publications.
References[edit]
keygenmusic.tkβ10-version
Supports .mod | .xm | .s3m | .it formats.
Thanks to keygenmusic.net for the music packs.
There is no illegal content. Just music from keygens
Contact me:

keygenmusic.tk{at}outlook.com
2016.02.05 - β10 - mod title, search by mod titles ...
2015.10.02 - update to September 2015 pack (keygenmusic.net)
2015.10.01 - β9
2015.09.07 - update to July 2015 pack (keygenmusic.net)
2015.06.05 - β8 - now playing in playlist
2015.06.04 - upd to April 2015 pack (keygenmusic.net)
2015.05.25 - β7
2015.05.18 - β6 - spectrum
2015.05.12 - β5 - favorites, link to song
2015.05.08 - β4 - optimizations
2015.05.04 - β2 - Shuffle on/off, song search
2015.04.30 - β1 - February 2015 music pack from keygenmusic.net
music from keygenmusic.net
#libs, tools etc#
chiptune2.js, libopenmpt, emscripten, jsTabs, baron, Sergio Camalich, wothke.ch/webaudio68, thanks to Darcula color scheme, Shuffle on/off switcher
Contact me:

keygenmusic.tk{at}outlook.com
What’s New in the Kings Edit 1.xx serial key or number?
Screen Shot
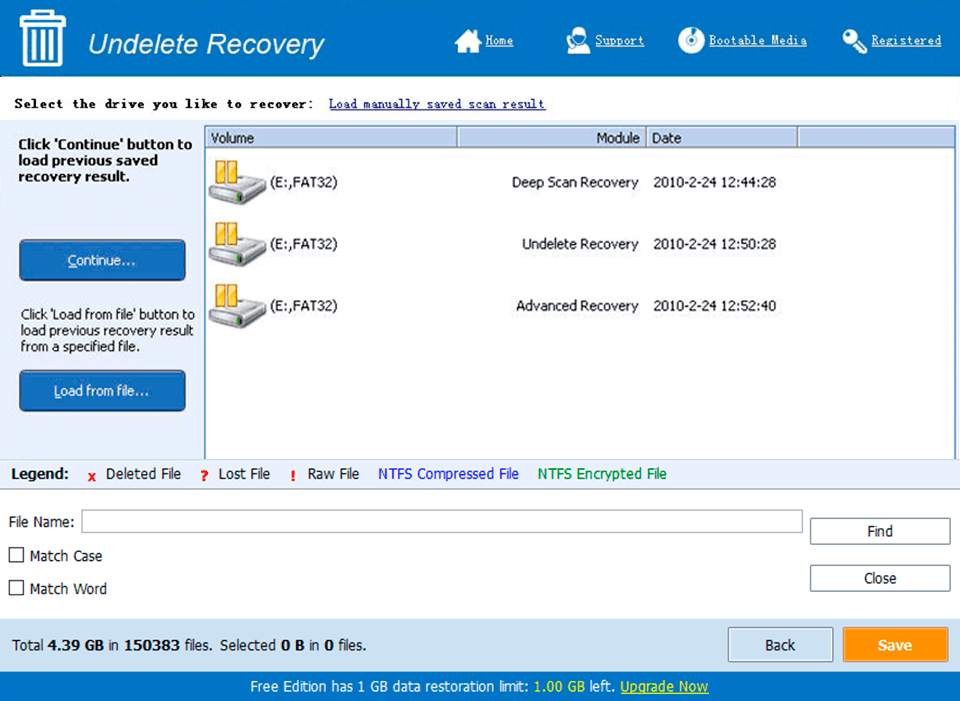
System Requirements for Kings Edit 1.xx serial key or number
- First, download the Kings Edit 1.xx serial key or number
-
You can download its setup from given links: