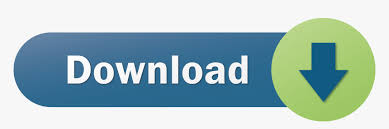
KnockOut 1.1 serial key or number
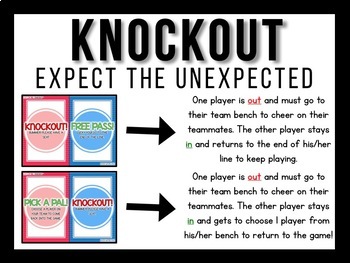
KnockOut 1.1 serial key or number
Myosin-X knockout is semi-lethal and demonstrates that myosin-X functions in neural tube closure, pigmentation, hyaloid vasculature regression, and filopodia formation
Abstract
Myosin-X (Myo10) is an unconventional myosin best known for its striking localization to the tips of filopodia. Despite the broad expression of Myo10 in vertebrate tissues, its functions at the organismal level remain largely unknown. We report here the generation of KO-first (Myo10tm1a/tm1a), floxed (Myo10tm1c/tm1c), and KO mice (Myo10tm1d/tm1d). Complete knockout of Myo10 is semi-lethal, with over half of homozygous KO embryos exhibiting exencephaly, a severe defect in neural tube closure. All Myo10 KO mice that survive birth exhibit a white belly spot, all have persistent fetal vasculature in the eye, and ~50% have webbed digits. Myo10 KO mice that survive birth can breed and produce litters of KO embryos, demonstrating that Myo10 is not absolutely essential for mitosis, meiosis, adult survival, or fertility. KO-first mice and an independent spontaneous deletion (Myo10m1J/m1J) exhibit the same core phenotypes. During retinal angiogenesis, KO mice exhibit a ~50% decrease in endothelial filopodia, demonstrating that Myo10 is required to form normal numbers of filopodia in vivo. The Myo10 mice generated here demonstrate that Myo10 has important functions in mammalian development and provide key tools for defining the functions of Myo10 in vivo.
Introduction
Myosin-X (Myo10) is an unconventional myosin expressed in most vertebrate tissues, including brain, testis, kidney, and endothelia1. Despite intense interest in its cellular and biophysical properties2,3, little is known regarding the organismal functions of Myo10. Mutant mice have been crucial for understanding the functions of other myosins and their roles in human disease, as illustrated by Myo5a in dilute lethal mice and human Griscelli syndrome4, Myo6 in Snell’s Waltzer mice and human nonsyndromic deafness5, Myo7a in Shaker-1 mice and human Usher 1b deaf-blindness syndrome6,7, and Myo15a in Shaker-2 mice and human deafness DFNB38,9. To fully define the functions of Myo10, it is thus essential to generate Myo10 knockout (KO) mice and determine their phenotype.
Myo10 is a member of a phylogenetically ancient group of myosins characterized by one or more MyTH4-FERM domains (Myosin Tail Homology 4 and band 4.1, Ezrin, Radixin, Merlin). The MyTH4-FERM myosins are strongly associated with protrusions based on actin bundles, such as filopodia, microvilli, and inner ear stereocilia10,11. Of the four MyTH4-FERM myosins expressed in humans, Myo7a is expressed in several tissues and localizes to inner ear stereocilia12, Myo7b is expressed in transporting epithelia and localizes to the tips of microvilli13, Myo15a is expressed in the inner ear and localizes to the tips of stereocilia14, and Myo10 is expressed in most cells and localizes to the tips of filopodia3.
The heavy chain of full-length mouse Myo10 is encoded by 41 exons that span 191 kb on chromosome 15. Myo10 contains 2062 aa (amino acids) that form a head, neck, and tail. The head domain binds to actin filaments and hydrolyzes ATP to generate force and movement3. The neck consists of a light chain binding domain with 3 IQ motifs, each of which binds to a calmodulin or calmodulin-like light chain. The tail domain begins with a stable α-helix15 followed by a short α-helical region that can dimerize by forming an anti-parallel coiled coil2,16. The remainder of the tail includes a PEST region, 3 Pleckstrin Homology (PH) domains, and a MyTH4-FERM domain1. The second PH domain binds to the important signaling lipid PI(3,4,5)P3, and activation of PI3 kinase is thought to recruit Myo10 monomers to the plasma membrane, triggering them to form mechanochemically active dimers17,18,19. The MyTH4 domain can bind to microtubules, allowing Myo10 to act as a motorized link between actin filaments and microtubules20,21,22. The FERM domain can bind to the cytoplasmic domains of several cell surface proteins, including β-integrins23 and the netrin receptors DCC (Deleted in Colorectal Cancer) and neogenin24. Neogenin is also a coreceptor for Bone Morphogenic Proteins (BMP)25, and Myo10 is upregulated by BMP and functions in BMP signaling26,27.
Although most cells and tissues appear to express only the ~237 kD full-length Myo10, neural stem cells, neurons, and astrocytes express both full-length and a ~165 kD, “headless” form of Myo1028,29. This work showed that use of an alternative transcription start site located ~8.6 kb into intron 19 generates a headless specific exon consisting entirely of 5′ UTR and that splicing this to exons 20–41 generates a transcript for headless Myo10. This headless transcript (corresponding to NCBI reference sequence XM_192774.2) encodes a protein expected to initiate at M644 and thus lack most of the motor domain, but is otherwise identical to full-length Myo10. The NCBI predicts that two other alternative transcription start sites are located ~13.9 and ~29.5 kb into intron 19 and generate two additional transcripts for headless Myo10 (XM_006520025.3 and XM_006520024.3). The first encodes a headless protein that is identical to the headless protein described above and corresponds to aa 644–2062 of full-length Myo10; the second is identical except that it adds a single M preceding aa 644–2062. Headless Myo10 does not induce filopodia and has been hypothesized to act as a natural dominant negative and/or as a scaffold that interacts with Myo10 binding partners such as PIP3, microtubules, and β-integrins29,30.
Full-length Myo10 is strongly associated with filopodia3, finger-like protrusions thought to function in migration, adhesion, and signaling31,32. In cell culture, overexpressing full-length Myo10 increases filopodia number, whereas inhibiting Myo10 decreases filopodia number33,34,35,36. Extremely faint particles of GFP-Myo10 within filopodia move rapidly towards the tip at rates of ~600 nm/s37,38, leading to the hypothesis that full-length Myo10 powers the intrafilopodial transport of candidate cargo molecules like integrins. In vitro studies with Myo10 indicate that it is specialized to move on parallel actin bundles and can move processively at rates of over ~600 nm/s2,39. Myo10 also functions in microtubule-dependent processes like spindle orientation20,40,41. Growing evidence indicates that Myo10 has important roles in cancer biology. Specifically, Myo10 is upregulated in several cancers, is a key component of invadopodia, and inhibiting it suppresses invasion42,43,44,45.
By generating mice with combined loss of full-length and headless Myo10, we show that complete loss of Myo10 is semi-lethal, with over half of null embryos exhibiting exencephaly. Myo10 null mice that survive birth exhibit several abnormalities, and demonstrate that Myo10 is required to form normal numbers of filopodia in vivo. Together this work shows that Myo10 has important functions in mammalian development in neural tube closure, pigmentation, and regression of the fetal vasculature of the eye.
Results
Generation of Myo10 KO-first, floxed, and KO mice
To generate mice that completely lack all forms of Myo10, we began by obtaining C57BL/6 derived embryonic stem cells from the Knockout Mouse Project (KOMP) that target exon 27 of Myo10 with a KO-first cassette (www.komp.org; Myo10tm1a(KOMP)Wtsi). The KO-first cassette contains a strong splice acceptor site and stop codon upstream of exon 27 that are expected to truncate full-length Myo10 and the three headless transcripts after aa 1190 (Fig. 1A,B). The KO-first cassette also contains an internal ribosomal entry site (IRES) upstream of lacZ to allow X-gal labeling of cells that express full-length or headless Myo10. Because the KO-first cassette is flanked by FRT sites, exposing the Myo10tm1a allele to Flp recombinase is expected to excise the KO-first cassette, which should restore Myo10 expression and generate the conditional Myo10tm1c allele. Because exon 27 is flanked by loxP sites, exposure of the Myo10tm1c allele to Cre recombinase is expected to delete exon 27 and generate a true genomic KO allele (Myo10tm1d).
To obtain germline transmission of the Myo10tm1a allele on a C57BL/6 background, the Myo10tm1a ES cells were injected into blastocysts and the resulting chimeras were bred with albino C57BL/6 mice. Offspring carrying the Myo10tm1a allele were crossed with C57BL/6 mice expressing FLPo recombinase to excise the KO-first cassette and generate the floxed Myo10tm1c allele on a C57BL/6 background. Myo10tm1c mice were then crossed with a global Cre-deleter strain (β-actin Cre) on a C57BL/6 background to delete exon 27 and generate the Myo10tm1d KO allele. Mice carrying the Myo10tm1d allele were then bred to one another and maintained as a germline KO on the C57BL/6 background. As expected, immunoblots of whole brain from P5 pups showed that control mice expressed both full-length and headless Myo10, while neither was detected in the Myo10tm1a/tm1a KO-first. Removal of the KO-first cassette produced the conditional Myo10tm1c/tm1c and restored expression of full-length and headless Myo10, while neither form of Myo10 was detected in the Myo10tm1d/tm1d KO (Fig. 1C).
Myo10 KO mice have ~60% incidence of exencephaly
When heterozygous Myo10+/tm1d mice were crossed with one another, pups were obtained at the ratio of 1 Myo10+/+: 2 Myo10+/tm1d: 0.4 Myo10tm1d/tm1d (Fig. 2A). This differs from the expected Mendelian ratio of 1:2:1 in that Myo10tm1d/tm1d null pups were obtained only ~40% as often as expected (30 Myo10tm1d/tm1d null pups versus 77 Myo10+/+ wild type). Myo10+/tm1d heterozygotes, however, were obtained at the expected 2:1 ratio relative to Myo10+/+ homozygotes and appeared indistinguishable from them, thus demonstrating that one copy of the Myo10 gene is sufficient for normal development. The lower than expected number of Myo10tm1d/tm1d pups led us to suspect that ~60% of Myo10tm1d/tm1d embryos might undergo embryonic or perinatal lethality and not survive to be counted as pups. To test this, we dissected pregnant Myo10+/tm1d (n = 4) and Myo10tm1d/tm1d (n = 2) mice that had been mated to Myo10tm1d/tm1d males and examined Myo10tm1d/tm1d embryos between E12.5 and E17.5. Strikingly, 15 of the 22 (68%) Myo10tm1d/tm1d embryos exhibited exencephaly (Fig. 2B), a severe defect in neural tube closure that is incompatible with survival after birth46. Because over half of Myo10tm1d/tm1d embryos were exencephalic, these results reveal that Myo10 has important functions in neural tube closure, and that combined loss of full-length and headless Myo10 is semi-lethal.
Myo10 KO mice have a white belly spot and a high frequency of syndactyly
We next investigated the phenotype of the ~40% of Myo10tm1d/tm1d mice that survived birth. The average weight at weaning (P21) was 7.6 g ± 2.1 for Myo10tm1d/tm1d (n = 9), which is slightly smaller than the 9.8 g ± 1.0 for Myo10+/+ (n = 4, p = 0.026) and 9.9 g ± 1.1 for Myo10+/tm1d (n = 9, p = 0.01). A similar difference was also observed at P28 (Supplementary Figure 1). KO pups were able to nurse, feed, and mature into adults. Strikingly, 100% of the Myo10tm1d/tm1d mice exhibited a white spot on the belly instead of the uniform black coat expected for C57BL/6 mice (Fig. 2C). Additional pigmentation defects, such as a white spot on the back, were observed in a few cases. The belly spot was noticeable as soon as black pigmentation developed elsewhere and provided a simple visual screen to confirm the PCR genotyping of Myo10 null mice. ~50% of Myo10tm1d/tm1d mice exhibited webbed digits, a form of syndactyly, with the webbed digits usually affecting the forelimb (Fig. 2D). Approximately 75% of Myo10tm1d/tm1d mice exhibited a visible kink near the tip of the tail (Fig. 2F). 53% of the homozygous Myo10tm1d/tm1d mice obtained were male, and Myo10tm1d/tm1d homozygotes were able to develop into mature adults that could mate with one another to produce Myo10tm1d/tm1d embryos and pups. This demonstrates that meiosis and mitosis can occur in the complete absence of Myo10, and that embryonic development can occur in the absence of maternal Myo10. Young adult Myo10tm1d/tm1d mice did not exhibit obvious movement defects like circling or shaking and responded to a hand clap by flicking their ears (Preyer reflex), indicating that hearing and balance are grossly intact at this age. The oldest Myo10tm1d/tm1d mice have survived over a year and a half without obvious morbidity or mortality. Together these results demonstrate that Myo10 has important roles in mammalian development, but is not strictly essential for adult survival or fertility.
Myo10 KO mice have persistent hyaloid vasculature
Dissection of eyes from adult mice followed by careful removal of the lens revealed that a black, pigmented mass was present bilaterally in all 11 Myo10tm1d/tm1d mice tested but none of 14 Myo10+/+orMyo10+/tm1d mice (Fig. 2E) The mass was typically attached to the optic disc by a thin strand and extended through the vitreous to form a plexus on the posterior surface of the lens. In some eyes, the retrolental mass appeared to have integrated into the retina and disrupted the retina’s normal morphology. The position and morphology of the retrolental mass indicate that it is persistent hyaloid vasculature. This condition is also known as persistent fetal vasculature (PFV) or persistent hyperplastic primary vitreous (PHPV), and results from a hyperplasia and persistence of a normally transient fetal vasculature that perfuses the vitreous during development47. The hyaloid vasculature originates at the optic disk as the stalk of the hyaloid artery and includes numerous branches, some of which extend through the periphery of the vitreous while others form a plexus on the posterior surface of the lens. The hyaloid vasculature in mouse begins forming at approximately E12.5 as the optic fissure closes, and is normally resorbed over the first few postnatal weeks as the retinal vasculature grows out onto the surface of the retina and matures. In humans, PFV is responsible for 5% of childhood blindness and is associated with increased incidence of several other ocular abnormalities including microphthalmia (a small eye)47. When a series of 11 Myo10tm1d/tm1d E12.5-E14.5 embryos were examined for major eye defects, 3/11 exhibited severe microphthalmia and 2/11 exhibited anophthalmia (absence of an eye).
To investigate the development of the persistent hyaloid vasculature, we dissected eyes from pups at P5, a time when the hyaloid vasculature would have just started to resorb. The retinal vasculature, on the other hand, would have begun angiogenesis at ~P0 by growing out from the optic disc and by P5 should extend approximately halfway across the retina48. Dissection revealed that a pigmented mass (Fig. 3A) was present bilaterally in 17 Myo10tm1d/tm1d mice, while no examples were present in any of the eyes from 12 control mice. Immunofluorescence with the endothelial cell marker PECAM-1 (CD31) showed that numerous long and relatively straight hyaloid vessels were present in the control eye at P5; as expected, the hyaloid vessels originated near the optic disk and extended branches towards the ciliary body (Fig. 3B). In the Myo10tm1d/tm1d retina, however, the hyaloid artery extended as a thickened stalk from the optic disk into a pigmented mass. The pigmented mass includes a plexus of blood vessels and pigment cells, with long and relatively straight hyaloid vessels extending outward from arms on the mass toward the ciliary body. The presence of the pigmented mass at P5 demonstrates that it forms and becomes pigmented prior to the major regression of hyaloid vessels in control eyes. Pigmented cells were not observed on either the stalk of the hyaloid artery or the hyaloid vessels extending towards the ciliary body, both of which represent potential pathways for pigment cell migration (Fig. 3B,D). Together these experiments demonstrate that a core element of the Myo10tm1d/tm1d phenotype is 100% penetrance of persistent hyaloid vasculature that is pigmented and bilateral.
Myo10 KO-first mice also exhibit a high incidence of exencephaly, a white belly spot, and retention of the hyaloid vasculature
In addition to determining the phenotype of the definitive germline KO mice, we also investigated the phenotype of the KO-first mice (Myo10tm1a/tm1a). Myo10+/tm1a heterozygotes appeared indistinguishable from controls, but when they were crossed to each other, pups were obtained at the ratio of 1 Myo10+/+: 1.5 Myo10+/tm1a: 0.45 Myo10tm1a/tm1a (20 Myo10+/+, 30 Myo10+/tm1a, 9 Myo10tm1a/tm1a; n = 9 litters). Because Myo10tm1a/tm1a homozygotes were obtained only 45% as often as Myo10+/+ homozygotes, these results again suggested that loss of Myo10 was leading to a high frequency of embryonic or perinatal lethality. Consistent with this, examination of Myo10tm1a/tm1a E12.5 embryos resulting from 2 homozygous crosses of Myo10tm1a/tm1a mice revealed that 50% (9/18) had exencephaly. Myo10tm1a/tm1a homozygotes that survived birth grew to adulthood and 100% had a white belly spot. A few also had additional pigmentation defects such as a white spot on the back. 100% of Myo10tm1a/tm1a mice whose eyes were dissected (5/5) had persistent hyaloid vasculature that was pigmented and bilateral. Approximately 50% of Myo10tm1a/tm1a mice had webbed digits and similar fraction had a kink near the tip of the tail. Importantly, when the KO-first mice were crossed with FLPo mice to delete the KO-first cassette, Myo10 expression was restored (Fig. 1C) and the resulting Myo10tm1c/tm1c mice appeared completely normal. This rescue of the KO-first phenotype provides strong genetic evidence that the phenotypes reported here are specifically due to disruption of Myo10.
Myo10 is required to form normal numbers of filopodia during retinal angiogenesis
Given in vitro studies indicating that Myo10 is a key component of filopodia3 we next sought to determine if Myo10 is required for filopodial formation in vivo. Therefore, we turned to the retinal vasculature, where endothelial tip cells generate numerous filopodia during angiogenesis as they grow out across the retina48,49. To visualize the endothelial cells and their filopodia, P5 retinas were stained with a PECAM-1 antibody (Fig. 4A). Low power views showed that the retinal vasculature grew similar distances across the retina in both control and Myo10tm1d/tm1d retinas (1.34 ± 0.11 mm for 9 controls and 1.25 ± 0.08 for 5 Myo10tm1d/tm1d, p = 0.09), demonstrating that angiogenic outgrowth can occur in the absence of Myo10. The vascular network, however, appeared to be less dense in Myo10tm1d/tm1d retinas, and quantification showed that the branches/mm2 decreased by over 30% (Fig. 4A,C). To determine if loss of Myo10 decreased filopodia, we collected Z-stacks of the vascular front at 60x and displayed these as maximum projections. This revealed that the endothelial cells in Myo10tm1d/tm1d retinas had far fewer filopodia than controls (Fig. 4B). Quantification showed a ~50% decrease in filopodia per mm along the vascular front (Fig. 4D), thus demonstrating that Myo10 is required to form normal numbers of filopodia in vivo.
Myo10m1J/m1J mice exhibit exencephaly, a white belly spot, and retention of the hyaloid vasculature
To conclusively define the phenotype resulting from loss of full-length and headless Myo10, we also examined mice carrying an independent mutation (Myo10m1J) that was recently listed along with 91 other new mouse mutations identified at The Jackson Laboratory50. The Myo10m1J mutation, which is on a C57BL/6 background, is due to a spontaneous 8 bp deletion (CGACGAGT) in exon 25 and produces a frame shift expected to truncate full-length Myo10 and all three headless transcripts following aa 948. The brief online description of the Myo10m1J mutation (http://www.informatics.jax.org/reference/J:214794) indicates that homozygotes exhibited a white belly spot. Syndactyly on the forelimbs was also noted, and persistent fetal vasculature was visible in the two mice whose eyes were examined. Homozygous Myo10m1J/m1J mice were listed as viable, but as having a decreased body size. However, no immunoblots were performed to confirm loss of Myo10 protein, no data was presented on the proportion of homozygotes obtained, and no mention was made of exencephaly.
Immunoblots of whole brain from a month-old Myo10m1J/m1J mouse show that neither full-length nor headless Myo10 was detected, demonstrating that the mutation results in complete loss of Myo10 (Fig. 5A). Myo10+/m1J heterozygotes appeared normal, but when they were mated to one another, pups were obtained in the ratio of 1 Myo10+/+: 2.3 Myo10+/m1J: 0.3 Myo10m1J/m1J (Fig. 5B). The much lower than expected number of Myo10m1J/m1J pups (14 Myo10m1J/m1J nulls versus 48 Myo10+/+) again suggested that loss of Myo10 leads a high level of embryonic or perinatal lethality. Analysis of embryos (E12.5-E17.5) from heterozygous matings revealed that 22/88 (25%) were Myo10m1J/m1J homozygotes, indicating that null embryos were present in the expected Mendelian percentage. Examination of the 22 null embryos revealed that 73% had exencephaly (Fig. 5C), 18% had craniorachischisis (a major failure of neural tube closure at the level of the hindbrain and spinal cord), and 9% had a gross developmental defect such as very small size. This demonstrates that a large fraction of Myo10m1J/m1J embryos have exencephaly and/or other defects that would be incompatible with survival past birth. Examples of fetuses undergoing resorption were also observed, and 64% of homozygous embryos exhibited blood in the amniotic fluid. Homozygous embryos appeared slightly smaller than controls, even when not affected by exencephaly or other major defects.
PMC
Discussion
Exon-specific knockout and knockin as complementary tools to assess the function of a splice variant protein
Here, we describe the first exon-specific knockout mice to assess the function of a muscle-specific splice variant of β1, the β1D integrin subunit. Because of the numerous biological functions of β1, the conventional knockout of the β1 gene is embryonically lethal already during gastrulation (Fässler et al. 1995; Stephens et al. 1995), so that the function of the β1D integrin cannot be assessed in these mice. To overcome this problem we have applied a genetic approach on the basis of the Cre–loxP system (Gu et al. 1994; DiSanto et al. 1995; Smith et al. 1995) by which exon D can be specifically deleted. This procedure did not interfere with the germ-line transmission of the mutated gene because chimeric and heterozygous mice were readily obtained. Importantly, the replacement of exon D by a loxP site did not interfere with either the transcription of the β1 gene or the stability or the splicing of the β1 pre-mRNA, as shown by the normal expression of the β1 mRNA in knockout mice (Fig. (Fig.2C).2C). Finally, translation of the mature RNA also appears to be normal because the total amount of the β1 proteins in muscles from knockout and control mice were similar (Fig. (Fig.22D).
In addition, we have also generated mice that express β1D, instead of β1A, by applying a knockin strategy. This method has been used previously to replace the mouse Engrailed gene En-1 by En-2 (Hanks et al. 1995). Here, we describe, for the first time, the knockin of an exon, replacing the normal mRNA by one encoding one of its splice variants. ES cells were transfected with a construct allowing the constitutive expression of β1D mRNA. Northern blot analysis of RNA demonstrated that the procedure did not affect the transcription of the β1 gene or the stability of the β1D mRNA, that is, the level of β1D in the ki/ki MEFs was the same as that of β1A in the +/+ MEFs (Fig. (Fig.5E).5E). Furthermore, the knockin procedure did not interfere with the germ-line transmission of the ki gene because the ratio of heterozygous mice was as expected.
In principle, these two complementary techniques, exon-specific knockout and knockin, should allow the activation and inactivation of any exon in any gene. Thus, it is now possible to study the role of splice variants in a genetically modified animal.
What does the knockout tell us about the function of β1D in muscles?
Considering results of previous studies that indicate that β1 plays an important role in muscle cell differentiation (Fässler et al. 1996), the organization of sarcomeric cytoarchitecture (Volk et al. 1990) and myogenesis (Yang et al. 1996), we were surprised that in our study the lack of β1D did not affect muscle formation and did not cause muscular degeneration or myopathy. A likely explanation for this unexpected finding is that loss of the function of β1D is compensated by β1A. The β1D isoform is very homologous to β1A; the two variants differ only by 13 amino acids of their cytoplasmic tails (van der Flier et al. 1995; Zhidkova et al. 1995; Belkin et al. 1996). Thus, the phenotype observed in the ko/ko mice is only the result of a different function of the β1D-specific amino acids (Fig. (Fig.4B),4B), rather than of the total β1 subunit.
In skeletal muscle, β1D is present at the myotendinous junction and at the costameres. These structures are the major junctions through which the skeletal muscle fiber is connected with the extracellular matrix and the tendon. In the heart, β1D is found at the intercalated discs that are the structure units for cell–cell contact of the cardiomyocyte (Belkin et al. 1996). In skeletal muscles, β1D is associated with the α7A and α7B subunits, whereas in heart it is only associated with α7B. Recent work has shown that the cytoplasmic domain of the βPS subunit, which is the β1 integrin-like subunit in Drosophila, is responsible and sufficient for targeting integrins to the termini of muscles in vivo (Martin-Bermudo and Brown 1996). From these observations, it seems that the cytoplasmic domain of β1D plays a role in the localization of integrins at these sites. However, in the β1D ko/ko mice β1A is localized at the same position as β1D in normal muscle, that is, intercalated discs, myotendinous junctions, and costameres, which excludes a specific role for β1D in targeting integrins to these sites. Furthermore, the data suggest that the association of β1A with sarcomeric protein and the actin network is normal and that the role of β1D is not different from that of β1A in the maintenance and the organization of the cytoarchitecture of the mature muscle cells. Finally, β1D ko/ko mice form normal mature muscles, excluding a specific role for β1D in myogenesis. Because β1D is highly enriched at the myotendinous junctions and in intercalated discs, it has been proposed that β1D provides stronger attachment at these sites than β1A (van der Flier et al. 1995; Belkin et al. 1996). Our data do not support this assumption because there are no histological or ultrastructural abnormalities in skeletal and cardiac muscles from ko/ko mice. We also did not observe an increased expression of the dystrophin/dystroglycan complex, which would be a possible compensatory response to a weakening of the attachment of the muscle to the extracellular matrix. Finally, the localization of the α2 laminin subunit appeared to be normal.
What could be the function of the β1D integrin? The production of isoforms by developmentally regulated alternative splicing of pre-mRNAs is a widespread phenomenon that represents an evolutionary strategy aimed at a subtle functional diversification. Consequently, defining the function of a splice variant protein might be more difficult than of a protein in a total gene knockout. Hence, to identify subtle defects, we analyzed our ko/ko mice further for functional alterations of the myocardium, because β1D is 5–10 times more strongly expressed in the heart than in skeletal muscles (van der Flier et al. 1995). In addition, the myocardium is subject to continuous mechanical stress. The amount of ANP is a reliable biological marker for the severity of functional and haemodynamic heart failure. ANP is a 28-amino-acid polypeptide hormone secreted by the heart in response to mechanical and neuroendocrine stimuli (Jarygin et al. 1994; Nakao et al. 1996). In early heart failure, ANP synthesis is increased in the ventricles in which it may play a key role in preserving a compensated state of asymptomatic heart dysfunction (Brandt et al. 1993). In humans and hamsters with congestive heart failure and in rats with aortic valve insufficiency, ANP mRNA levels are increased three- to fivefold, respectively, in the ventricles (Brooks et al. 1988; Morita et al. 1990). In addition, ANP mRNA is also increased sixfold in ventricles of rats with spontaneous biventricular hypertrophy (Lee et al. 1988). The finding that in our ko/ko male mice the ANP mRNA and the βMHC protein levels are increased up to sixfold and by 25%, respectively, is consistent with a ventricular dysfunction despite the lack of any histological abnormalities or hypertrophic responses.
Why does the absence of β1D lead to an increased synthesis of ANP? In the heart, ANP is secreted in response to stretch induced by blood pressure that is detected by mainly two factors, that is, pressure-sensitive channel proteins (Erdos et al. 1991), and most likely integrins, which may function as mechanotransducers (Nakao et al. 1996; Shyy and Chien 1997). In light of our results, we speculate that β1D acts as a mechanotransducer with a reduced sensitivity to the shear force as compared with β1A. We suggest that on stretching of muscle cells, the signals elicited by β1D and β1A are different. Support for this assumption is derived from the observation that in cultured cells β1A and β1D transmit different signals after crosslinking with antibodies (Belkin et al. 1996). Thus, it is conceivable that in our β1D ko/ko mice, the β1A-mediated reactivity to blood pressure is perturbed, inducing increased synthesis of ANP. This increase in ANP synthesis seems to be sex linked because it was observed in 100% of the male and 0% of the female mice. The reason for this is unknown, but we suspect that male mice have a slightly higher blood pressure than female mice. In addition, studies have reported that female rats are more protected against muscle damage following exercise than male rats. This protective effect is probably the result of female sex hormones, particularly estrogens (Tiidus et al. 1995).
What does the knockin tell us about the functions of β1A in development?
The most striking of the defects that we observed was the shortening of the first branchial arches that resulted in an underdeveloped lower mandible (micrognathia) and an open neural tube. Defective closures, both in the brain (anencephaly) and in the lower spine (myelomeningcele), were evident and, in some cases, were accompanied by an unusual kinking of the back and tail, reminiscent of that described in FN ko/ko and α5 ko/ko embryos at 8.5 dpc (George et al. 1993; Yang et al. 1993). FN, which normally surrounds the neural tube, was absent in the region of spina bifida, which suggests that its absence is related to this defect. Poor elongation of the embryonic axis in the β1D ki/ki embryos accompanied by normal neural tube elongation, however, is a more likely cause of its kinking, as has also been suggested in FN ko/ko embryos. Unexpectedly, the α5 integrin subunit, which we had assumed would bind FN to cells surrounding the neural tube, was normally distributed in the mutant embryos. It is conceivable that α5β1D transmits different signals or interacts with other cystokeletal proteins than α5β1A and, thus, affects the assembly of FN in ki/ki embryos. This hypothesis is supported by the work of Wu et al. (1995) who have shown that integrin postoccupancy events, requiring the cytoplasmic domain of the β chain and an intact cytoskeleton are needed for FN fibrillogenesis. Although other FN-binding integrins may partially compensate for α5β1-induced matrix assembly during development (Yang and Hynes 1996), their ability to do so is less efficient as compared with that of α5β1, as was demonstrated with ES cells (Wennerberg et al. 1996), which explains the altered distribution of FN in our ki/ki embryos. Other striking defects that we observed were an abnormally smooth head with poorly expanded brain cavities, in some cases accompanied by a virtual lack of choroid plexus in the roof of the fourth ventricle. We suspect that an altered migratory potential of neuroepithelial cells may underly these alterations. It is also tempting to speculate that the migratory capacity of the neural crest cells, the major migratory cell population at these mid-gestational stages, is also reduced, because they are an important component of the first branchial arch, in which we observe a defect, and because these cells are thought to be guided to their destination by various extracellular matrix (ECM) molecules, including FN (Morrison-Graham et al. 1992
Disruption of thyroid hormone activation in type 2 deiodinase knockout mice causes obesity with glucose intolerance and liver steatosis only at thermoneutrality.
REFERENCES
What’s New in the KnockOut 1.1 serial key or number?
Screen Shot
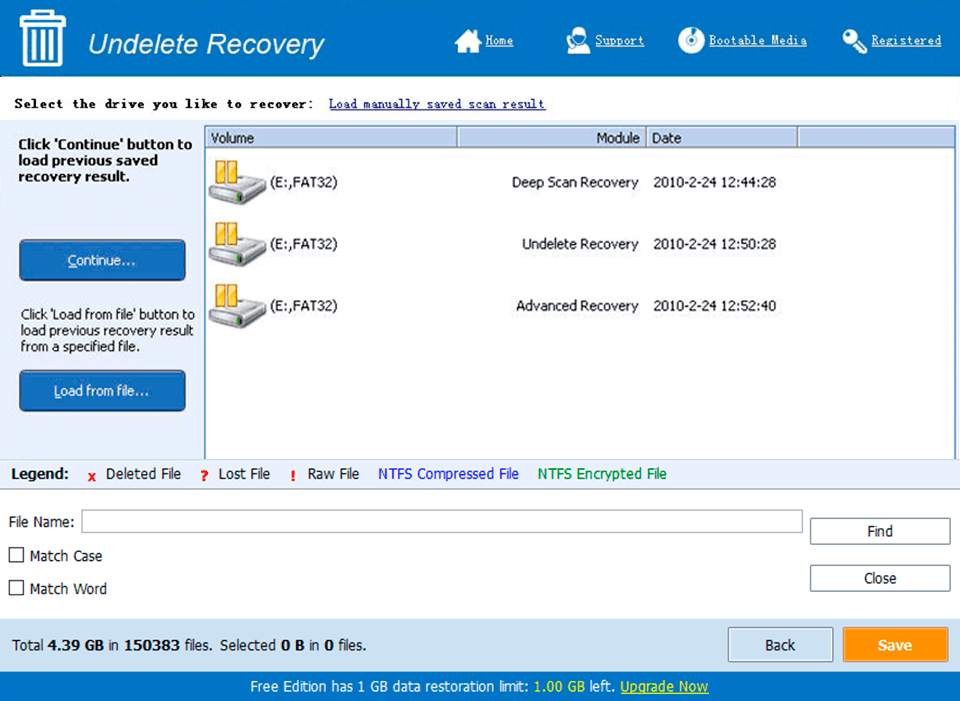
System Requirements for KnockOut 1.1 serial key or number
- First, download the KnockOut 1.1 serial key or number
-
You can download its setup from given links: