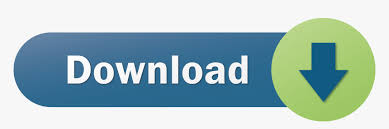
Periodic Table 2002 v1.2.1 serial key or number

Periodic Table 2002 v1.2.1 serial key or number
The Periodic Table I
As 2019 has been declared the International Year of the Periodic Table, it is appropriate that Structure and Bonding marks this anniversary with two special volumes.
In 1869 Dmitri Ivanovitch Mendeleev first proposed his periodic table of the elements. He is given the major credit for proposing the conceptual framework used by chemists to systematically inter-relate the chemical properties of the elements. However, the concept of periodicity evolved in distinct stages and was the culmination of work by other chemists over several decades. For example, Newland’s Law of Octaves marked an important step in the evolution of the periodic system since it represented the first clear statement that the properties of the elements repeated after intervals of 8. Mendeleev’s predictions demonstrated in an impressive manner how the periodic table could be used to predict the occurrence and properties of new elements. Not all of his many predictions proved to be valid, but the discovery of scandium, gallium and germanium represented sufficient vindication of its utility and they cemented its enduring influence. Mendeleev’s periodic table was based on the atomic weights of the elements and it was another 50 years before Moseley established that it was the atomic number of the elements, that was the fundamental parameter and this led to the prediction of further elements.
Some have suggested that the periodic table is one of the most fruitful ideas in modern science and that it is comparable to Darwin’s theory of evolution by natural selection, proposed at approximately the same time. There is no doubt that the periodic table occupies a central position in chemistry. In its modern form it is reproduced in most undergraduate inorganic textbooks and is present in almost every chemistry lecture room and classroom.
This first volume provides chemists with an account of the historical development of the Periodic Table and an overview of how the Periodic Table has evolved over the last 150 years. It also illustrates how it has guided the research programmes of some distinguished chemists.
Keywords
- 1.Inorganic Chemistry LaboratoryUniversity of OxfordOxfordUK
Bibliographic information
Chalcogen
The chalcogens (/ˈkælkədʒɪnz/) are the chemical elements in group 16 of the periodic table. This group is also known as the oxygen family. It consists of the elements oxygen (O), sulfur (S), selenium (Se), tellurium (Te), and the radioactive element polonium (Po). The chemically uncharacterized synthetic elementlivermorium (Lv) is predicted to be a chalcogen as well.[1] Often, oxygen is treated separately from the other chalcogens, sometimes even excluded from the scope of the term "chalcogen" altogether, due to its very different chemical behavior from sulfur, selenium, tellurium, and polonium. The word "chalcogen" is derived from a combination of the Greek word khalkόs (χαλκός) principally meaning copper (the term was also used for bronze/brass, any metal in the poetic sense, ore or coin),[2] and the Latinised Greek word genēs, meaning born or produced.[3][4]
Sulfur has been known since antiquity, and oxygen was recognized as an element in the 18th century. Selenium, tellurium and polonium were discovered in the 19th century, and livermorium in 2000. All of the chalcogens have six valence electrons, leaving them two electrons short of a full outer shell. Their most common oxidation states are −2, +2, +4, and +6. They have relatively low atomic radii, especially the lighter ones.[5]
Lighter chalcogens are typically nontoxic in their elemental form, and are often critical to life, while the heavier chalcogens are typically toxic.[1] All of the naturally occurring chalcogens have some role in biological functions, either as a nutrient or a toxin. Selenium is an important nutrient (among others as a building block of selenocysteine) but is also commonly toxic.[6] Tellurium often has unpleasant effects (although some organisms can use it), and polonium (especially the isotopepolonium-210) is always harmful as a result of its radioactivity.
Sulfur has more than 20 allotropes, oxygen has nine, selenium has at least eight, polonium has two, and only one crystal structure of tellurium has so far been discovered. There are numerous organic chalcogen compounds. Not counting oxygen, organic sulfur compounds are generally the most common, followed by organic selenium compounds and organic tellurium compounds. This trend also occurs with chalcogen pnictides and compounds containing chalcogens and carbon group elements.
Oxygen is generally obtained by separation of air into nitrogen and oxygen. Sulfur is extracted from oil and natural gas. Selenium and tellurium are produced as byproducts of copper refining. Polonium and livermorium are most available in particle accelerators. The primary use of elemental oxygen is in steelmaking. Sulfur is mostly converted into sulfuric acid, which is heavily used in the chemical industry.[6] Selenium's most common application is glassmaking. Tellurium compounds are mostly used in optical disks, electronic devices, and solar cells. Some of polonium's applications are due to its radioactivity.[1]
Properties[edit]
Atomic and physical[edit]
Chalcogens show similar patterns in electron configuration, especially in the outermost shells, where they all have the same number of valence electrons, resulting in similar trends in chemical behavior:
Z | Element | No. of electrons/shell |
---|---|---|
8 | Oxygen | 2, 6 |
16 | Sulfur | 2, 8, 6 |
34 | Selenium | 2, 8, 18, 6 |
52 | Tellurium | 2, 8, 18, 18, 6 |
84 | Polonium | 2, 8, 18, 32, 18, 6 |
116 | Livermorium | 2, 8, 18, 32, 32, 18, 6 (predicted)[7] |
Element | Melting point (°C)[5] | Boiling point (°C)[5] | Density at STP (g/cm3)[5] |
---|---|---|---|
Oxygen | −219 | −183 | 0.00143 |
Sulfur | 120 | 445 | 2.07 |
Selenium | 221 | 685 | 4.3 |
Tellurium | 450 | 988 | 6.24 |
Polonium | 254 | 962 | 9.2 |
Livermorium | 220(predicted) | 800(predicted) | 14(predicted)[7] |
All chalcogens have six valence electrons. All of the solid, stable chalcogens are soft[8] and do not conduct heat well.[5]Electronegativity decreases towards the chalcogens with higher atomic numbers. Density, melting and boiling points, and atomic and ionic radii[9] tend to increase towards the chalcogens with higher atomic numbers.[5]
Isotopes[edit]
Out of the six known chalcogens, one (oxygen) has an atomic number equal to a nuclear magic number, which means that their atomic nuclei tend to have increased stability towards radioactive decay.[10] Oxygen has three stable isotopes, and 14 unstable ones. Sulfur has four stable isotopes, 20 radioactive ones, and one isomer. Selenium has six observationally stable or nearly stable isotopes, 26 radioactive isotopes, and 9 isomers. Tellurium has eight stable or nearly stable isotopes, 31 unstable ones, and 17 isomers. Polonium has 42 isotopes, none of which are stable.[11] It has an additional 28 isomers.[1] In addition to the stable isotopes, some radioactive chalcogen isotopes occur in nature, either because they are decay products, such as 210Po, because they are primordial, such as 82Se, because of cosmic rayspallation, or via nuclear fission of uranium. Livermorium isotopes 290Lv through 293Lv have been discovered; the most stable livermorium isotope is 293Lv, which has a half-life of 0.061 seconds.[1][12]
Among the lighter chalcogens (oxygen and sulfur), the most neutron-poor isotopes undergo proton emission, the moderately neutron-poor isotopes undergo electron capture or β+ decay, the moderately neutron-rich isotopes undergo β− decay, and the most neutron rich isotopes undergo neutron emission. The middle chalcogens (selenium and tellurium) have similar decay tendencies as the lighter chalcogens, but their isotopes do not undergo proton emission and some of the most neutron-deficient isotopes of tellurium undergo alpha decay. Polonium's isotopes tend to decay with alpha or beta decay.[13] Isotopes with nuclear spins are more common among the chalcogens selenium and tellurium than they are with sulfur.[14]
Allotropes[edit]


Oxygen's most common allotrope is diatomic oxygen, or O2, a reactive paramagnetic molecule that is ubiquitous to aerobic organisms and has a blue color in its liquid state. Another allotrope is O3, or ozone, which is three oxygen atoms bonded together in a bent formation. There is also an allotrope called tetraoxygen, or O4,[16] and six allotropes of solid oxygen including "red oxygen", which has the formula O8.[17]
Sulfur has over 20 known allotropes, which is more than any other element except carbon.[18] The most common allotropes are in the form of eight-atom rings, but other molecular allotropes that contain as few as two atoms or as many as 20 are known. Other notable sulfur allotropes include rhombic sulfur and monoclinic sulfur. Rhombic sulfur is the more stable of the two allotropes. Monoclinic sulfur takes the form of long needles and is formed when liquid sulfur is cooled to slightly below its melting point. The atoms in liquid sulfur are generally in the form of long chains, but above 190° Celsius, the chains begin to break down. If liquid sulfur above 190°Celsius is frozen very rapidly, the resulting sulfur is amorphous or "plastic" sulfur. Gaseous sulfur is a mixture of diatomic sulfur (S2) and 8-atom rings.[19]
Selenium has at least eight distinct allotropes.[20] The gray allotrope, commonly referred to as the "metallic" allotrope, despite not being a metal, is stable and has a hexagonal crystal structure. The gray allotrope of selenium is soft, with a Mohs hardness of 2, and brittle. Four other allotropes of selenium are metastable. These include two monoclinic red allotropes and two amorphous allotropes, one of which is red and one of which is black.[21] The red allotrope converts to the red allotrope in the presence of heat. The gray allotrope of selenium is made from spirals on selenium atoms, while one of the red allotropes is made of stacks of selenium rings (Se8).[1][dubious – discuss]
Tellurium is not known to have any allotropes,[22] although its typical form is hexagonal. Polonium has two allotropes, which are known as α-polonium and β-polonium.[23] α-polonium has a cubic crystal structure and converts to the rhombohedral β-polonium at 36 °C.[1]
The chalcogens have varying crystal structures. Oxygen's crystal structure is monoclinic, sulfur's is orthorhombic, selenium and tellurium have the hexagonal crystal structure, while polonium has a cubic crystal structure.[5][6]
Chemical[edit]
Oxygen, sulfur, and selenium are nonmetals, and tellurium is a metalloid, meaning that its chemical properties are between those of a metal and those of a nonmetal.[6] It is not certain whether polonium is a metal or a metalloid. Some sources refer to polonium as a metalloid,[1][24] although it has some metallic properties. Also, some allotropes of selenium display characteristics of a metalloid,[25] even though selenium is usually considered a nonmetal. Even though oxygen is a chalcogen, its chemical properties are different from those of other chalcogens. One reason for this is that the heavier chalcogens have vacant d-orbitals. Oxygen's electronegativity is also much higher than those of the other chalcogens. This makes oxygen's electric polarizability several times lower than those of the other chalcogens.[14]
For covalent bonding a chalcogen may accept two electrons according to the octet rule, leaving two lone pairs. When an atom forms two single bonds, they form an angle between 90° and 120°. In 1+ cations, such as H
3O+
, a chalcogen forms three molecular orbitals arranged in a trigonal pyramidal fashion and one lone pair. Double bonds are also common in chalcogen compounds, for example in chalcogenates (see below).
The oxidation number of the most common chalcogen compounds with positive metals is −2. However the tendency for chalcogens to form compounds in the −2 state decreases towards the heavier chalcogens.[26] Other oxidation numbers, such as −1 in pyrite and peroxide, do occur. The highest formal oxidation number is +6.[5] This oxidation number is found in sulfates, selenates, tellurates, polonates, and their corresponding acids, such as sulfuric acid.
Oxygen is the most electronegative element except for fluorine, and forms compounds with almost all of the chemical elements, including some of the noble gases. It commonly bonds with many metals and metalloids to form oxides, including iron oxide, titanium oxide, and silicon oxide. Oxygen's most common oxidation state is −2, and the oxidation state −1 is also relatively common.[5] With hydrogen it forms water and hydrogen peroxide. Organic oxygen compounds are ubiquitous in organic chemistry.
Sulfur's oxidation states are −2, +2, +4, and +6. Sulfur-containing analogs of oxygen compounds often have the prefix thio-. Sulfur's chemistry is similar to oxygen's, in many ways. One difference is that sulfur-sulfur double bonds are far weaker than oxygen-oxygen double bonds, but sulfur-sulfur single bonds are stronger than oxygen-oxygen single bonds.[27] Organic sulfur compounds such as thiols have a strong specific smell, and a few are utilized by some organisms.[1]
Selenium's oxidation states are −2, +4, and +6. Selenium, like most chalcogens, bonds with oxygen.[1] There are some organic selenium compounds, such as selenoproteins. Tellurium's oxidation states are −2, +2, +4, and +6.[5] Tellurium forms the oxides tellurium monoxide, tellurium dioxide, and tellurium trioxide.[1] Polonium's oxidation states are +2 and +4.[5]

2O) is the most familiar chalcogen-containing compound.
There are many acids containing chalcogens, including sulfuric acid, sulfurous acid, selenic acid, and telluric acid. All hydrogen chalcogenides are toxic except for water.[28][29] Oxygen ions often come in the forms of oxide ions (O2−
), peroxide ions (O2−
2), and hydroxide ions (OH−
). Sulfur ions generally come in the form of sulfides (S2−
), sulfites (SO2−
3), sulfates (SO2−
4), and thiosulfates (S
2O2−
3). Selenium ions usually come in the form of selenides (Se2−
) and selenates (SeO2−
4). Tellurium ions often come in the form of tellurates (TeO2−
4).[5] Molecules containing metal bonded to chalcogens are common as minerals. For example, pyrite (FeS2) is an iron ore, and the rare mineral calaverite is the ditelluride (Au, Ag)Te2.
Although all group 16 elements of the periodic table, including oxygen, can be defined as chalcogens, oxygen and oxides are usually distinguished from chalcogens and chalcogenides. The term chalcogenide is more commonly reserved for sulfides, selenides, and tellurides, rather than for oxides.[30][31][32]
Except for polonium, the chalcogens are all fairly similar to each other chemically. They all form X2− ions when reacting with electropositive metals.[26]
Sulfide minerals and analogous compounds produce gases upon reaction with oxygen.[33]
Compounds[edit]
With halogens[edit]
Chalcogens also form compounds with halogens known as chalcohalides. Such compounds are known as chalcogen halides.[dubious – discuss] The majority of simple chalcogen halides are well-known and widely used as chemical reagents. However, more complicated chalcogen halides, such as sulfenyl, sulfonyl, and sulfuryl halides, are less well known to science. Out of the compounds consisting purely of chalcogens and halogens, there are a total of 13 chalcogen fluorides, nine chalcogen chlorides, eight chalcogen bromides, and six chalcogen iodides that are known.[dubious – discuss] The heavier chalcogen halides often have significant molecular interactions. Sulfur fluorides with low valences are fairly unstable and little is known about their properties.[dubious – discuss] However, sulfur fluorides with high valences, such as sulfur hexafluoride, are stable and well-known. Sulfur tetrafluoride is also a well-known sulfur fluoride. Certain selenium fluorides, such as selenium difluoride, have been produced in small amounts. The crystal structures of both selenium tetrafluoride and tellurium tetrafluoride are known. Chalcogen chlorides and bromides have also been explored. In particular, selenium dichloride and sulfur dichloride can react to form organic selenium compounds. Dichalcogen dihalides, such as Se2Cl2 also are known to exist. There are also mixed chalcogen-halogen compounds. These include SeSX, with X being chlorine or bromine.[dubious – discuss] Such compounds can form in mixtures of sulfur dichloride and selenium halides. These compounds have been fairly recently structurally characterized, as of 2008. In general, diselenium and disulfur chlorides and bromides are useful chemical reagents. Chalcogen halides with attached metal atoms are soluble in organic solutions.[dubious – discuss] One example of such a compound is MoS2Cl3. Unlike selenium chlorides and bromides, selenium iodides have not been isolated, as of 2008, although it is likely that they occur in solution. Diselenium diiodide, however, does occur in equilibrium with selenium atoms and iodine molecules. Some tellurium halides with low valences, such as Te2Cl2 and Te2Br2, form polymers when in the solid state. These tellurium halides can be synthesized by the reduction of pure tellurium with superhydride and reacting the resulting product with tellurium tetrahalides. Ditellurium dihalides tend to get less stable as the halides become lower in atomic number and atomic mass. Tellurium also forms iodides with even fewer iodine atoms than diiodies. These include TeI and Te2I. These compounds have extended structures in the solid state. Halogens and chalcogens can also form halochalcogenate anions.[31]
Organic[edit]
Alcohols, phenols and other similar compounds contain oxygen. However, in thiols, selenols and tellurols; sulfur, selenium, and tellurium replace oxygen. Thiols are better known than selenols or tellurols. Thiols are the most stable chalcogenols and tellurols are the least stable, being unstable in heat or light. Other organic chalcogen compounds include thioethers, selenoethers and telluroethers. Some of these, such as dimethyl sulfide, diethyl sulfide, and dipropyl sulfide are commercially available. Selenoethers are in the form of R2Se or RSeR. Telluroethers such as dimethyl telluride are typically prepared in the same way as thioethers and selenoethers. Organic chalcogen compounds, especially organic sulfur compounds, have the tendency to smell unpleasant. Dimethyl telluride also smells unpleasant,[34] and selenophenol is renowned for its "metaphysical stench".[35] There are also thioketones, selenoketones, and telluroketones. Out of these, thioketones are the most well-studied with 80% of chalcogenoketones papers being about them. Selenoketones make up 16% of such papers and telluroketones make up 4% of them. Thioketones have well-studied non-linear electric and photophysic properties. Selenoketones are less stable than thioketones and telluroketones are less stable than selenoketones. Telluroketones have the highest level of polarity of chalcogenoketones.[31]
With metals[edit]
Elemental chalcogens react with certain lanthanide compounds to form lanthanide clusters rich in chalcogens.[dubious – discuss]Uranium(IV) chalcogenol compounds also exist. There are also transition metal chalcogenols which have potential to serve as catalysts and stabilize nanoparticles.[31]
There is a very large number of metal chalcogenides. One of the more recent discoveries in this group of compounds is Rb2Te. There are also compounds in which alkali metals and transition metals such as the fourth period transition metals except for copper and zinc. In highly metal-rich metal chalcogenides, such as Lu7Te and Lu8Te have domains of the metal's crystal lattice containing chalcogen atoms. While these compounds do exist, analogous chemicals that contain lanthanum, praseodymium, gadolinium, holmium, terbium, or ytterbium have not been discovered, as of 2008. The boron group metals aluminum, gallium, and indium also form bonds to chalcogens. The Ti3+ ion forms chalcogenide dimers such as TiTl5Se8. Metal chalcogenide dimers also occur as lower tellurides, such as Zr5Te6.[31]
With pnictogens[edit]
Compounds with chalcogen-phosphorus bonds have been explored for more than 200 years. These compounds include unsophisticated phosphorus chalcogenides as well as large molecules with biological roles and phosphorus-chalcogen compounds with metal clusters. These compounds have numerous applications, including strike-anywhere matches and quantum dots. A total of 130,000 compounds with at least one phosphorus-sulfur bond, 6000 compounds with at least one phosphorus-selenium bond, and 350 compounds with at least one phosphorus-tellurium bond have been discovered.[citation needed] The decrease in the number of chalcogen-phosphorus compounds further down the periodic table is due to diminishing bond strength. Such compounds tend at least one phosphorus atom in the center, surrounded by four chalcogens and side chains. However, some phosphorus-chalcogen compounds also contain hydrogen (such as secondary phosphine chalcogenides) or nitrogen (such as dichalcogenoimidodiphosphates). Phosphorus selenides are typically harder to handle that phosphorus sulfides, and compounds in the form PxTey have not been discovered. Chalcogens also bond with other pnictogens, such as arsenic, antimony, and bismuth. Heavier chalcogen pnictides tend to form ribbon-like polymers instead of individual molecules. Chemical formulas of these compounds include Bi2S3 and Sb2Se3. Ternary chalcogen pnictides are also known. Examples of these include P4O6Se and P3SbS3. salts containing chalcogens and pnictogens also exist. Almost all chalcogen pnictide salts are typically in the form of [PnxE4x]3−, where Pn is a pnictogen and E is a chalcogen.[dubious – discuss] Tertiary phosphines can react with chalcogens to form compounds in the form of R3PE, where E is a chalcogen. When E is sulfur, these compounds are relatively stable, but they are less so when E is selenium or tellurium. Similarly, secondary phosphines can react with chalcogens to form secondary phosphine chalcogenides. However, these compounds are in a state of equilibrium with chalcogenophosphinous acid. Secondary phosphine chalcogenides are weak acids.[31] Binary compounds consisting of antimony or arsenic and a chalcogen. These compounds tend to be colorful and can be created by a reaction of the constituent elements at temperatures of 500 to 900 °C (932 to 1,652 °F).[36]
Other[edit]
Chalcogens form single bonds and double bonds with other carbon group elements than carbon, such as silicon, germanium, and tin. Such compounds typically form from a reaction of carbon group halides and chalcogenol salts or chalcogenol bases. Cyclic compounds with chalcogens, carbon group elements, and boron atoms exist, and occur from the reaction of boron dichalcogenates and carbon group metal halides. Compounds in the form of M-E, where M is silicon, germanium, or tin, and E is sulfur, selenium or tellurium have been discovered. These form when carbon group hydrides react or when heavier versions of carbenes react.[dubious – discuss] Sulfur and tellurium can bond with organic compounds containing both silicon and phosphorus.[31]
All of the chalcogens form hydrides. In some cases this occurs with chalcogens bonding with two hydrogen atoms.[1] However tellurium hydride and polonium hydride are both volatile and highly labile.[37] Also, oxygen can bond to hydrogen in a 1:1 ratio as in hydrogen peroxide, but this compound is unstable.[26]
Chalcogen compounds form a number of interchalcogens. For instance, sulfur forms the toxic sulfur dioxide and sulfur trioxide.[26] Tellurium also forms oxides. There are some chalcogen sulfides as well. These include selenium sulfide, an ingredient in some shampoos.[6]
Since 1990, a number of borides with chalcogens bonded to them have been detected. The chalcogens in these compounds are mostly sulfur, although some do contain selenium instead. One such chalcogen boride consists of two molecules of dimethyl sulfide attached to a boron-hydrogen molecule. Other important boron-chalcogen compounds include macropolyhedral systems. Such compounds tend to feature sulfur as the chalcogen. There are also chalcogen borides with two, three, or four chalcogens. Many of these contain sulfur but some, such as Na2B2Se7 contain selenium instead.[38]
History[edit]
Early discoveries[
Selenium
![]() | |||||||||||||||
Selenium | |||||||||||||||
---|---|---|---|---|---|---|---|---|---|---|---|---|---|---|---|
Pronunciation | /sɪˈliːniəm/ (sə-LEE-nee-əm) | ||||||||||||||
Appearance | black, red, and gray (not pictured) allotropes | ||||||||||||||
Standard atomic weight Ar, std(Se) | 78.971(8)[1] | ||||||||||||||
Selenium in the periodic table | |||||||||||||||
Atomic number(Z) | 34 | ||||||||||||||
Group | group 16 (chalcogens) | ||||||||||||||
Period | period 4 | ||||||||||||||
Block | p-block | ||||||||||||||
Element category | Reactive nonmetal, sometimes considered a metalloid | ||||||||||||||
Electron configuration | [Ar] 3d10 4s2 4p4 | ||||||||||||||
Electrons per shell | 2, 8, 18, 6 | ||||||||||||||
Physical properties | |||||||||||||||
Phaseat STP | solid | ||||||||||||||
Melting point | 494 K (221 °C, 430 °F) | ||||||||||||||
Boiling point | 958 K (685 °C, 1265 °F) | ||||||||||||||
Density (near r.t.) | gray: 4.81 g/cm3 alpha: 4.39 g/cm3 vitreous: 4.28 g/cm3 | ||||||||||||||
when liquid (at m.p.) | 3.99 g/cm3 | ||||||||||||||
Critical point | 1766 K, 27.2 MPa | ||||||||||||||
Heat of fusion | gray: 6.69 kJ/mol | ||||||||||||||
Heat of vaporization | 95.48 kJ/mol | ||||||||||||||
Molar heat capacity | 25.363 J/(mol·K) | ||||||||||||||
Vapor pressure
| |||||||||||||||
Atomic properties | |||||||||||||||
Oxidation states | −2, −1, +1,[2]+2, +3, +4, +5, +6 (a strongly acidic oxide) | ||||||||||||||
Electronegativity | Pauling scale: 2.55 | ||||||||||||||
Ionization energies |
| ||||||||||||||
Atomic radius | empirical: 120 pm | ||||||||||||||
Covalent radius | 120±4 pm | ||||||||||||||
Van der Waals radius | 190 pm | ||||||||||||||
![]() | |||||||||||||||
Other properties | |||||||||||||||
Natural occurrence | primordial | ||||||||||||||
Crystal structure | hexagonal![]() | ||||||||||||||
Speed of sound thin rod | 3350 m/s (at 20 °C) | ||||||||||||||
Thermal expansion | amorphous: 37 µm/(m·K) (at 25 °C) | ||||||||||||||
Thermal conductivity | amorphous: 0.519 W/(m·K) | ||||||||||||||
Magnetic ordering | diamagnetic[3] | ||||||||||||||
Magnetic susceptibility | −25.0·10−6 cm3/mol (298 K)[4] | ||||||||||||||
Young's modulus | 10 GPa | ||||||||||||||
Shear modulus | 3.7 GPa | ||||||||||||||
Bulk modulus | 8.3 GPa | ||||||||||||||
Poisson ratio | 0.33 | ||||||||||||||
Mohs hardness | 2.0 | ||||||||||||||
Brinell hardness | 736 MPa | ||||||||||||||
CAS Number | 7782-49-2 | ||||||||||||||
History | |||||||||||||||
Naming | after Selene, Greek goddess of the moon | ||||||||||||||
Discovery and first isolation | Jöns Jakob Berzelius and Johann Gottlieb Gahn (1817) | ||||||||||||||
Main isotopes of selenium | |||||||||||||||
![]() | references |
Selenium is a chemical element with the symbolSe and atomic number 34. It is a nonmetal (more rarely considered a metalloid) with properties that are intermediate between the elements above and below in the periodic table, sulfur and tellurium, and also has similarities to arsenic. It rarely occurs in its elemental state or as pure ore compounds in the Earth's crust. Selenium—from Ancient Greekσελήνη (selḗnē) "Moon" – was discovered in 1817 by Jöns Jacob Berzelius, who noted the similarity of the new element to the previously discovered tellurium (named for the Earth).
Selenium is found in metal sulfide ores, where it partially replaces the sulfur. Commercially, selenium is produced as a byproduct in the refining of these ores, most often during production. Minerals that are pure selenide or selenate compounds are known but rare. The chief commercial uses for selenium today are glassmaking and pigments. Selenium is a semiconductor and is used in photocells. Applications in electronics, once important, have been mostly replaced with silicon semiconductor devices. Selenium is still used in a few types of DC power surge protectors and one type of fluorescentquantum dot.
Selenium salts are toxic in large amounts, but trace amounts are necessary for cellular function in many organisms, including all animals. Selenium is an ingredient in many multivitamins and other dietary supplements, including infant formula. It is a component of the antioxidant enzymes glutathione peroxidase and thioredoxin reductase (which indirectly reduce certain oxidized molecules in animals and some plants). It is also found in three deiodinase enzymes, which convert one thyroid hormone to another. Selenium requirements in plants differ by species, with some plants requiring relatively large amounts and others apparently requiring none.[5]
Characteristics[edit]
Physical properties[edit]

Selenium forms several allotropes that interconvert with temperature changes, depending somewhat on the rate of temperature change. When prepared in chemical reactions, selenium is usually an amorphous, brick-red powder. When rapidly melted, it forms the black, vitreous form, usually sold commercially as beads.[6] The structure of black selenium is irregular and complex and consists of polymeric rings with up to 1000 atoms per ring. Black Se is a brittle, lustrous solid that is slightly soluble in CS2. Upon heating, it softens at 50 °C and converts to gray selenium at 180 °C; the transformation temperature is reduced by presence of halogens and amines.[7]
The red α, β, and γ forms are produced from solutions of black selenium by varying the evaporation rate of the solvent (usually CS2). They all have relatively low, monoclinic crystal symmetries and contain nearly identical puckered Se8 rings with different arrangements, as in sulfur. The packing is most dense in the α form. In the Se8 rings, the Se-Se distance is 233.5 pm and Se-Se-Se angle is 105.7° . Other selenium allotropes may contain Se6 or Se7 rings.[7]
The most stable and dense form of selenium is gray and has a hexagonal crystal lattice consisting of helical polymeric chains, where the Se-Se distance is 237.3 pm and Se-Se-Se angle is 130.1° . The minimum distance between chains is 343.6 pm. Gray Se is formed by mild heating of other allotropes, by slow cooling of molten Se, or by condensing Se vapor just below the melting point. Whereas other Se forms are insulators, gray Se is a semiconductor showing appreciable photoconductivity. Unlike the other allotropes, it is insoluble in CS2.[7] It resists oxidation by air and is not attacked by nonoxidizing acids. With strong reducing agents, it forms polyselenides. Selenium does not exhibit the changes in viscosity that sulfur undergoes when gradually heated.[6][8]
Optical properties[edit]
Owing to its use as a photoconductor in flat-panel x-ray detectors (see below), the optical properties of amorphous selenium (α-Se) thin films have been the subject of intense research.[9][10][11]
Isotopes[edit]
Selenium has seven naturally occurring isotopes. Five of these, 74Se, 76Se, 77Se, 78Se, 80Se, are stable, with 80Se being the most abundant (49.6% natural abundance). Also naturally occurring is the long-lived primordial radionuclide82Se, with a half-life of 9.2×1019 years.[12] The non-primordial radioisotope 79Se also occurs in minute quantities in uranium ores as a product of nuclear fission. Selenium also has numerous unstable synthetic isotopes ranging from 64Se to 95Se; the most stable are 75Se with a half-life of 119.78 days and 72Se with a half-life of 8.4 days.[12] Isotopes lighter than the stable isotopes primarily undergo beta plus decay to isotopes of arsenic, and isotopes heavier than the stable isotopes undergo beta minus decay to isotopes of bromine, with some minor neutron emission branches in the heaviest known isotopes.
Isotope | Nature | Origin | Half-life |
---|---|---|---|
74Se | Primordial | Stable | |
76Se | Primordial | Stable | |
77Se | Primordial | Fission product | Stable |
78Se | Primordial | Fission product | Stable |
79Se | Trace | Fission product | 327000 yr[13][14] |
80Se | Primordial | Fission product | Stable |
82Se | Primordial | Fission product* | ~1020 yr[12][a] |
Chemical compounds[edit]
Selenium compounds commonly exist in the oxidation states −2, +2, +4, and +6.
Chalcogen compounds[edit]
Selenium forms two oxides: selenium dioxide (SeO2) and selenium trioxide (SeO3). Selenium dioxide is formed by the reaction of elemental selenium with oxygen:[6]
- Se8 + 8 O2 → 8 SeO2

It is a polymeric solid that forms monomeric SeO2 molecules in the gas phase. It dissolves in water to form selenous acid, H2SeO3. Selenous acid can also be made directly by oxidizing elemental selenium with nitric acid:[15]
- 3 Se + 4 HNO3 + H2O → 3 H2SeO3 + 4 NO
Unlike sulfur, which forms a stable trioxide, selenium trioxide is thermodynamically unstable and decomposes to the dioxide above 185 °C:[6][15]
- 2 SeO3 → 2 SeO2 + O2 (ΔH = −54 kJ/mol)
Selenium trioxide is produced in the laboratory by the reaction of anhydrouspotassium selenate (K2SeO4) and sulfur trioxide (SO3).[16]
Salts of selenous acid are called selenites. These include silver selenite (Ag2SeO3) and sodium selenite (Na2SeO3).
Hydrogen sulfide reacts with aqueous selenous acid to produce selenium disulfide:
- H2SeO3 + 2 H2S → SeS2 + 3 H2O
Selenium disulfide consists of 8-membered rings. It has an approximate composition of SeS2, with individual rings varying in composition, such as Se4S4 and Se2S6. Selenium disulfide has been used in shampoo as an antidandruff agent, an inhibitor in polymer chemistry, a glass dye, and a reducing agent in fireworks.[15]
Selenium trioxide may be synthesized by dehydrating selenic acid, H2SeO4, which is itself produced by the oxidation of selenium dioxide with hydrogen peroxide:[17]
- SeO2 + H2O2 → H2SeO4
Hot, concentrated selenic acid can react with gold to form gold(III) selenate.[18]
Halogen compounds[edit]
Iodides of selenium are not well known. The only stable chloride is selenium monochloride (Se2Cl2), which might be better known as selenium(I) chloride; the corresponding bromide is also known. These species are structurally analogous to the corresponding disulfur dichloride. Selenium dichloride is an important reagent in the preparation of selenium compounds (e.g. the preparation of Se7). It is prepared by treating selenium with sulfuryl chloride (SO2Cl2).[19] Selenium reacts with fluorine to form selenium hexafluoride:
- Se8 + 24 F2 → 8 SeF6
In comparison with its sulfur counterpart (sulfur hexafluoride), selenium hexafluoride (SeF6) is more reactive and is a toxic pulmonary irritant.[20] Some of the selenium oxyhalides, such as selenium oxyfluoride (SeOF2) and selenium oxychloride (SeOCl2) have been used as specialty solvents.[6]
Selenides[edit]
Analogous to the behavior of other chalcogens, selenium forms hydrogen selenide, H2Se. It is a strongly odiferous, toxic, and colorless gas. It is more acidic than H2S. In solution it ionizes to HSe−. The selenide dianion Se2− forms a variety of compounds, including the minerals from which selenium is obtained commercially. Illustrative selenides include mercury selenide (HgSe), lead selenide (PbSe), zinc selenide (ZnSe), and copper indium gallium diselenide (Cu(Ga,In)Se2). These materials are semiconductors. With highly electropositive metals, such as aluminium, these selenides are prone to hydrolysis:[6]
- Al2Se3 + 3 H2O → Al2O3 + 3 H2Se
Alkali metal selenides react with selenium to form polyselenides, Se2−
n, which exist as chains.
Other compounds[edit]
Tetraselenium tetranitride, Se4N4, is an explosive orange compound analogous to tetrasulfur tetranitride (S4N4).[6][21][22] It can be synthesized by the reaction of selenium tetrachloride (SeCl4) with [((CH
3)
3Si)
2N]
2Se.[23]
Selenium reacts with cyanides to yield selenocyanates:[6]
- 8 KCN + Se8 → 8 KSeCN
Organoselenium compounds[edit]
Selenium, especially in the II oxidation state, forms stable bonds to carbon, which are structurally analogous to the corresponding organosulfur compounds. Especially common are selenides (R2Se, analogues of thioethers), diselenides (R2Se2, analogues of disulfides), and selenols (RSeH, analogues of thiols). Representatives of selenides, diselenides, and selenols include respectively selenomethionine, diphenyldiselenide, and benzeneselenol. The sulfoxide in sulfur chemistry is represented in selenium chemistry by the selenoxides (formula RSe(O)R), which are intermediates in organic synthesis, as illustrated by the selenoxide elimination reaction. Consistent with trends indicated by the double bond rule, selenoketones, R(C=Se)R, and selenaldehydes, R(C=Se)H, are rarely observed.[24]
History[edit]
Selenium (Greek σελήνη selene meaning "Moon") was discovered in 1817 by Jöns Jacob Berzelius and Johan Gottlieb Gahn.[25] Both chemists owned a chemistry plant near Gripsholm, Sweden, producing sulfuric acid by the lead chamber process. The pyrite from the Falun Mine created a red precipitate in the lead chambers which was presumed to be an arsenic compound, so the pyrite's use to make acid was discontinued. Berzelius and Gahn wanted to use the pyrite and they also observed that the red precipitate gave off a smell like horseradish when burned. This smell was not typical of arsenic, but a similar odor was known from tellurium compounds. Hence, Berzelius's first letter to Alexander Marcet stated that this was a tellurium compound. However, the lack of tellurium compounds in the Falun Mine minerals eventually led Berzelius to reanalyze the red precipitate, and in 1818 he wrote a second letter to Marcet describing a newly found element similar to sulfur and tellurium. Because of its similarity to tellurium, named for the Earth, Berzelius named the new element after the Moon.[26][27]
In 1873, Willoughby Smith found that the electrical resistance of grey selenium was dependent on the ambient light.[28][29] This led to its use as a cell for sensing light. The first commercial products using selenium were developed by Werner Siemens in the mid-1870s. The selenium cell was used in the photophone developed by Alexander Graham Bell in 1879. Selenium transmits an electric current proportional to the amount of light falling on its surface. This phenomenon was used in the design of light meters and similar devices. Selenium's semiconductor properties found numerous other applications in electronics.[30][31][32] The development of selenium rectifiers began during the early 1930s, and these replaced copper oxide rectifiers because they were more efficient.[33][34][35] These lasted in commercial applications until the 1970s, following which they were replaced with less expensive and even more efficient silicon rectifiers.
Selenium came to medical notice later because of its toxicity to industrial workers. Selenium was also recognized as an important veterinary toxin, which is seen in animals that have eaten high-selenium plants. In 1954, the first hints of specific biological functions of selenium were discovered in microorganisms by biochemist, Jane Pinsent.[36][37] It was discovered to be essential for mammalian life in 1957.[38][39] In the 1970s, it was shown to be present in two independent sets of enzymes. This was followed by the discovery of selenocysteine in proteins. During the 1980s, selenocysteine was shown to be encoded by the codon UGA. The recoding mechanism was worked out first in bacteria and then in mammals (see SECIS element).[40]
Occurrence[edit]
Native (i.e., elemental) selenium is a rare mineral, which does not usually form good crystals, but, when it does, they are steep rhombohedra or tiny acicular (hair-like) crystals.[41] Isolation of selenium is often complicated by the presence of other compounds and elements.
Selenium occurs naturally in a number of inorganic forms, including selenide, selenate, and selenite, but these minerals are rare. The common mineral selenite is not a selenium mineral, and contains no selenite ion, but is rather a type of gypsum (calcium sulfate hydrate) named like selenium for the moon well before the discovery of selenium. Selenium is most commonly found as an impurity, replacing a small part of the sulfur in sulfide ores of many metals.[42][43]
In living systems, selenium is found in the amino acids selenomethionine, selenocysteine, and methylselenocysteine. In these compounds, selenium plays a role analogous to that of sulfur. Another naturally occurring organoselenium compound is dimethyl selenide.[44][45]
Certain soils are selenium-rich, and selenium can be bioconcentrated by some plants. In soils, selenium most often occurs in soluble forms such as selenate (analogous to sulfate), which are leached into rivers very easily by runoff.[42][43] Ocean water contains significant amounts of selenium.[46][47]
Anthropogenic sources of selenium include coal burning, and the mining and smelting of sulfide ores.[48]
Production[edit]
Selenium is most commonly produced from selenide in many sulfideores, such as those of copper, nickel, or lead. Electrolytic metal refining is particularly productive of selenium as a byproduct, obtained from the anode mud of copper refineries. Another source was the mud from the lead chambers of sulfuric acid plants, a process that is no longer used. Selenium can be refined from these muds by a number of methods. However, most elemental selenium comes as a byproduct of refining copper or producing sulfuric acid.[49][50] Since its invention, solvent extraction and electrowinning (SX/EW) production of copper produces an increasing share of the worldwide copper supply.[51] This changes the availability of selenium because only a comparably small part of the selenium in the ore is leached with the copper.[52]
Industrial production of selenium usually involves the extraction of selenium dioxide from residues obtained during the purification of copper. Common production from the residue then begins by oxidation with sodium carbonate to produce selenium dioxide, which is mixed with water and acidified to form selenous acid (oxidation step). Selenous acid is bubbled with sulfur dioxide (reduction step) to give elemental selenium.[53][54]
About 2,000 tonnes of selenium were produced in 2011 worldwide, mostly in Germany (650 t), Japan (630 t), Belgium (200 t), and Russia (140 t), and the total reserves were estimated at 93,000 tonnes. These data exclude two major producers: the United States and China. A previous sharp increase was observed in 2004 from $4–$5 to $27/lb. The price was relatively stable during 2004–2010 at about US$30 per pound (in 100 pound lots) but increased to $65/lb in 2011. The consumption in 2010 was divided as follows: metallurgy – 30%, glass manufacturing – 30%, agriculture – 10%, chemicals and pigments – 10%, and electronics – 10%. China is the dominant consumer of selenium at 1,500–2,000 tonnes/year.[55]
Applications[edit]
Fertilizers[edit]
Researchers found that application of selenium fertilizer to lettuce crops decreased the accumulation of lead and cadmium. Peaches and pears given a foliar selenium spray contained higher levels selenium and also stayed firm and ripe longer when in storage. In low doses, selenium has shown a beneficial effect on plant resistance to various environmental stress factors including drought, UV-B, soil salinity, and cold or hot temperatures. However, it can damage plants at higher doses.[56]
Manganese electrolysis[edit]
During the electrowinning of manganese, the addition of selenium dioxide decreases the power necessary to operate the electrolysis cells. China is the largest consumer of selenium dioxide for this purpose. For every tonne of manganese, an average 2 kg selenium oxide is used.[55][57]
Glass production[edit]
The largest commercial use of Se, accounting for about 50% of consumption, is for the production of glass. Se compounds confer a red color to glass. This color cancels out the green or yellow tints that arise from iron impurities typical for most glass. For this purpose, various selenite and selenate salts are added. For other applications, a red color may be desired, produced by mixtures of CdSe and CdS.[58]
Alloys[edit]
Selenium is used with bismuth in brasses to replace more toxic lead. The regulation of lead in drinking water applications such as in the US with the Safe Drinking Water Act of 1974, made a reduction of lead in brass necessary. The new brass is marketed under the name EnviroBrass.[59] Like lead and sulfur, selenium improves the machinability of steel at concentrations around 0.15%.[60][61] Selenium produces the same machinability improvement in copper alloys.[62]
Lithium–selenium batteries[edit]
What’s New in the Periodic Table 2002 v1.2.1 serial key or number?
Screen Shot
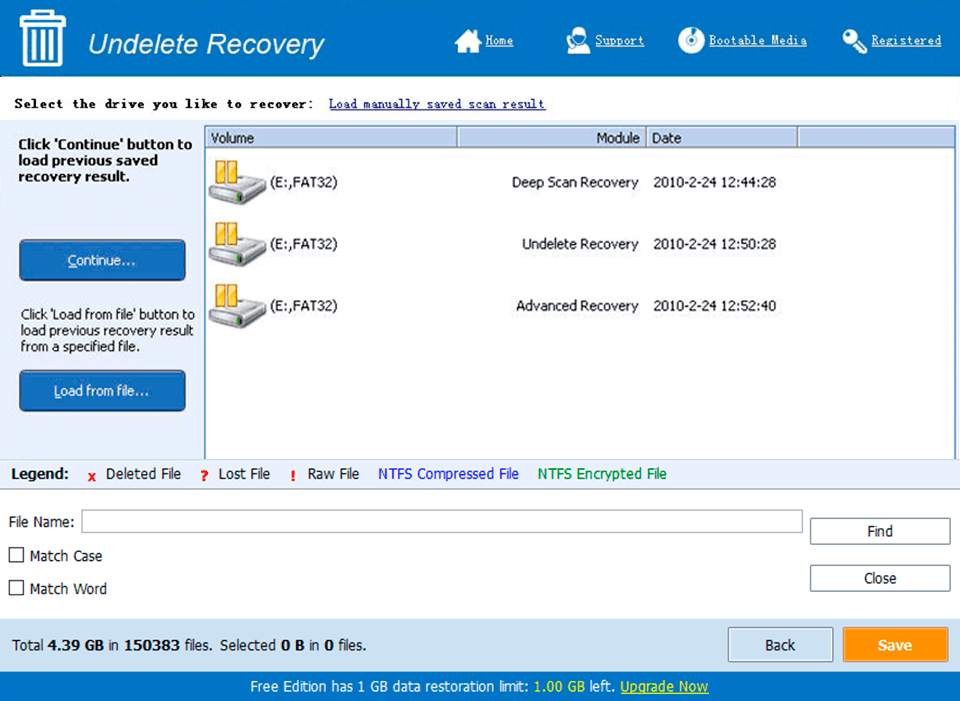
System Requirements for Periodic Table 2002 v1.2.1 serial key or number
- First, download the Periodic Table 2002 v1.2.1 serial key or number
-
You can download its setup from given links: